Using Synthetic Biology and pClone Red for Authentic Research on Promoter Function: Introductory Biology (identifying new promoters)
Published online:
Abstract
Students often memorize the definition of a transcriptional promoter but fail to fully understand the critical role promoters play in gene expression. This laboratory lesson allows students to conduct original research by identifying and characterizing promoters found in prokaryotes. Students start with primary literature, design and clone a short promoter, and test how well their promoter works. This laboratory lesson is an easy way for faculty with limited time and budgets to give their students access to real research in the context of traditional teaching labs that meet once a week for under three hours. The pClone Red Introductory Biology lesson uses synthetic biology methods and makes cloning so simple that we have 100% success rates with first year students. Students use a database to archive their promoter sequences and the performance of the promoter under standard conditions. The database permits synthetic biology researchers around the world to find a promoter that suits their needs and compare relative levels of transcription. The core methodology in this lesson is identical to the core methodology in the companion Genetics Lesson by Eckdahl and Campbell. The methods are reproduced in both lessons for the benefit of readers. The two CourseSource lessons provide the detailed information needed to reproduce the pedagogical research results published in CBE - Life Sciences Education by Campbell et al., 2014.
Citation
Campbell, A.M. and Eckdahl, T.T. 2015. Using Synthetic Biology and pClone Red for Authentic Research on Promoter Function: Introductory Biology (identifying new promoters). CourseSource. https://doi.org/10.24918/cs.2015.2
Society Learning Goals
Genetics
- Molecular biology of gene function
- How is genetic information expressed so it affects an organism's structure and function?
- Gene Expression and Regulation
- How do genes and genomes control changes in an organism's structure and function throughout its life cycle?
- Genetic variation
- How do different types of mutations affect genes and the corresponding mRNAs and proteins?
- Genetics of model organisms
- How do the results of molecular genetic studies in model organisms help us understand aspects of human genetics and genetic diseases?
- Methods & Tools in Genetics
- What experimental methods are commonly used to analyze gene structure and gene expression?
Science Process Skills
- Process of Science
- Pose testable questions and hypotheses to address gaps in knowledge
- Plan, evaluate, and implement scientific investigations
- Interpret, evaluate, and draw conclusions from data
- Construct explanations and make evidence-based arguments about the natural world
- Address novel questions through authentic research experiences
- Modeling/ Developing and Using Models
- Recognize the important roles that scientific models, of many different types (conceptual, mathematical, physical, etc.), play in predicting and communicating biological phenomena
- Make inferences and solve problems using models and simulations
- Build and evaluate models of biological systems
- Quantitative Reasoning/ Using Mathematics and Computational Thinking
- Apply the tools of graphing, statistics, and data science to analyze biological data
- Communication and Collaboration
- Share ideas, data, and findings with others clearly and accurately
Lesson Learning Goals
- Students understand how genes are regulated at the transcriptional level by internal and external conditions.
- Students will know how cells with the same genome can produce different proteins.
- Students will demonstrate how cell genomes can be manipulated through experimentation to alter function.
- Students will know how microorganisms serve as good model organisms for fundamental processes.
Lesson Learning Objectives
- Describe how cells can produce proteins at the right time and correct amount.
- Diagram how a repressor works to reduce transcription.
- Diagram how an activator works to increase transcription.
- Identify a new promoter from literature and design a method to clone it and test its function.
- Successfully and safely manipulate DNA and Escherichia coli for ligation and transformation experiments.
- Design an experiment to verify a new promoter has been cloned into a destination vector.
- Design an experiment to measure the strength of a promoter.
- Analyze data showing reporter protein produced and use the data to assess promoter strength.
- Define type IIs restriction enzymes.
- Distinguish between type II and type IIs restriction enzymes.
- Explain how Golden Gate Assembly (GGA) works.
- Measure the relative strength of a promoter compared to a standard promoter.
Article Context
Course
Article Type
Course Level
Bloom's Cognitive Level
Vision and Change Core Competencies
Vision and Change Core Concepts
Class Type
Class Size
Audience
Lesson Length
Pedagogical Approaches
Principles of How People Learn
Assessment Type
INTRODUCTION
One of the core concepts covered in just about every biology course is information storage and flow (1). A key concept in information flow is that promoters are key genetic elements that determine the conditions under which a gene will be transcribed and how much mRNA will be produced. We have been teaching for over twenty years and noticed that students could recite this definition of a promoter, but many were unable to relate the definition to cell functions. Students were often surprised to learn that mutations in promoters can result in new phenotypes or diseases. Therefore, we developed a new lab lesson that would give our students extensive, hands-on experience with promoters that they designed based on prior information from their textbook or primary literature (2). This lesson is for Introductory Biology and is closely associated with a companion lesson in Genetics (3). In addition to our goal of increased understanding of promoter structure and function, we wanted our students to conduct real research rather than simply demonstrate a known outcome. Conducting research improves science education while also increasing the number and diversity of scientifically literate citizens (4-9). We drew on our years of experience in mentoring undergraduates in synthetic biology research to accomplish both goals simultaneously (10-16).
Synthetic biology is the use of engineering principles, molecular cloning methods, and mathematical modeling to design and construct biological parts, devices, and systems with applications in energy, medicine, and technology. Synthetic biology is a new area of research that is amenable to undergraduate and high school students (17-21). Although our experience is with undergraduates, the pClone Red Introductory Biology lesson would also be appropriate for high school AP Biology courses, particularly for the newly designed AP program (22,23).
We find that, to really understand promoters, students need to study a promoter in the lab. In our lab lesson, students start by designing a promoter and making a prediction about its function, then they clone and test their promoter. For practical considerations, we limit students to promoter designs that are no more than 60 basepairs (bp) long because the company that supplies our oligonucleotides charges more per base for synthesis over 60 bases. Because it is very difficult to measure promoters in absolute units (e.g., number of mRNA molecules per cell), we provide students with a positive control RFP expression device (number J04450) that uses a very well characterized promoter (Plac; part number R0010) and have them calculate the relative strength of their promoter compared to Plac which is 200 bp long. One benefit of using Plac is that many classes also cover the lac operon. Using a familiar promoter reinforces concepts learned during class time.
Students taking the first year introductory biology course at Davidson are charged with finding a bacterial promoter described in the literature. They search PubMed and Google Scholar for open access papers that provide the DNA sequence as well as experimental test conditions. Students may choose a promoter that is negatively regulated by a repressor, or positively regulated by an activator, or one that is constitutive, meaning that it is not regulated. They are not allowed to choose common promoters such as Plac, Ptac, Pbad, Ptet, etc.
Once the promoters are designed, oligonucleotides that will form the 60nt double-stranded DNA promoter sequence are ordered and returned within three business days. When the two oligos are annealed, they produce unique sticky ends that ensure directional cloning which we have verified multiple times. The key to this lesson is the pClone Red plasmid (part number J119137) we designed and built (Figure 1; (24).

Figure 1. Diagram showing the key parts of the receiving plasmid pClone Red. A GFP gene (promoter, RBS and GFP coding) are oriented from right to left. The promoter is flanked by outwards-facing Bsa I restriction sites. When the promoter is cut out, the sticky ends (white boxes) allow a new promoter to be ligated and initiate transcription to the right of RBS and RFP coding.
"Red" refers to the common reporter red fluorescent protein (RFP) that is included in the plasmid. If unaltered pClone Red is transformed into cells, the cells appear green because they contain a GFP expression cassette of a "backward" green fluorescent protein (GFP) gene and a "backward" (right to left in Figure 1) promoter (PlacIQ1; part number K091112). This backward promoter is located between GFP and the "forward" (left to right in Figure 1) RFP coding sequences on the plasmid. Using a new cloning method that is extremely easy, called Golden Gate Assembly (GGA; (22,23,25,26), students replace the backward PlacIQ1 promoter in pClone Red with their experimental promoter, in a "forward" orientation. GGA employs type IIS restriction enzymes that allows students to simultaneously remove the backward promoter, generate two distinct sticky ends, and directionally ligate their forward promoter in its place. GGA eliminates GFP transcription driven by the "backward" promoter while cloning the "forward" experimental promoter. If the experimental promoter is functional, it will initiate transcription of RFP (Figure 2). If the experimental promoter is non-functional, neither GFP nor RFP will be expressed.
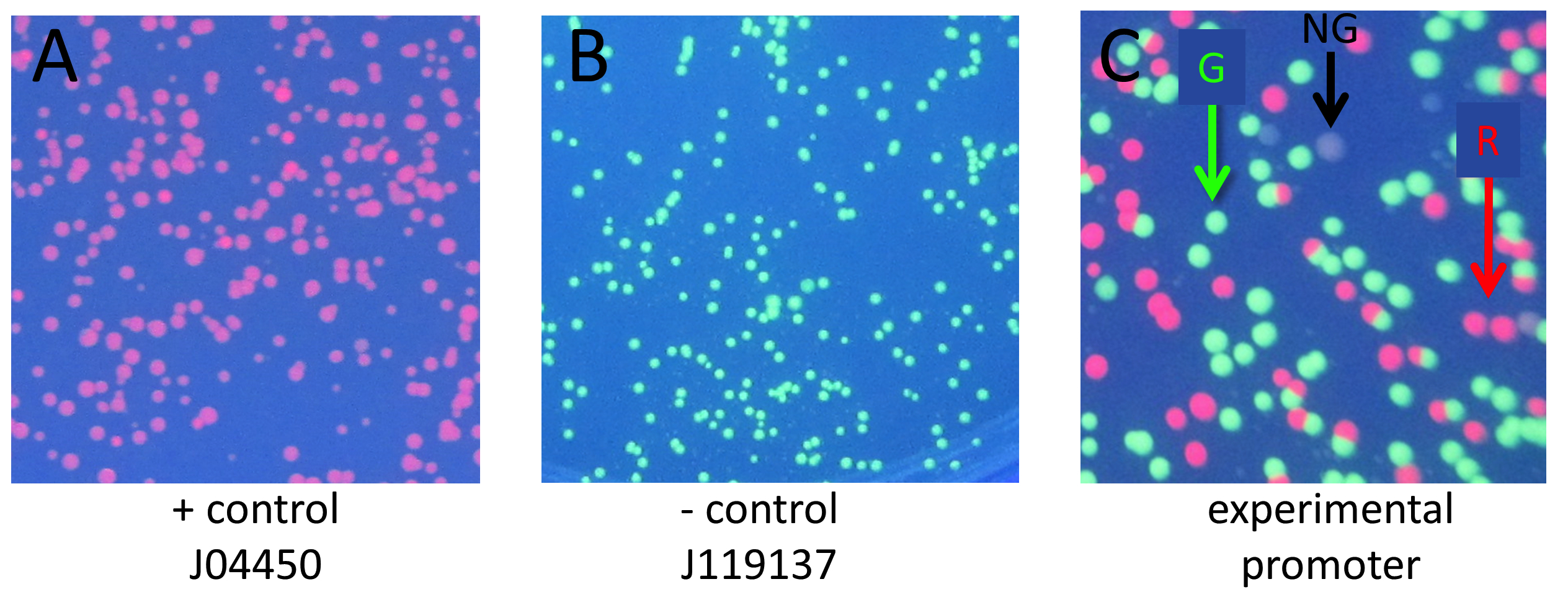
Figure 2. Close up photographs showing + control, - control and experimental plates. A) Positive control colonies containing Plac+RBS+RFP to produce only red colonies. B) Negative control colonies contain receiving plasmid pClone Red but no new promoter so colonies are mostly green with some colorless colonies. C) Experimental promoter cloned into pClone Red shows all three colony colors as indicated by colored arrows (R = red, G = green, NG = not green).
Students who attempt to clone a promoter can see three possible colors of colonies. Green colonies still contain the original backward promoter. Red colonies contain a functional experimental promoter. Colorless colonies contain non-functional experimental promoters.
The removal of the old promoter and insertion of the new promoter with GGA takes place in a single tube in one hour. Transformation of E. coli takes about 15 minutes. Students can easily perform the entire process in a normal laboratory session. After students have transformed and plated the bacterial cells, each lab group prepares to quantify the RFP produced by the transformed cells using a fluorometer (24). Students can easily visualize red colonies using UV light or white light and pick only red colonies. If no colonies appear red, they can pick multiple non-green colonies and screen for red using the fluorometer.
Intended Audience
Introductory biology laboratory course for potential majors, first-year students
Learning Time
Minimum of four weeks in lab, plus time for oral and written presentations.
Pre-requisite student knowledge
This lesson teaches students to pipet small volumes and work sterilely with bacteria. They need to know DNA base pairing and have learned about transcription, though we cover this material in class the same weeks when students are designing their promoters. This laboratory lesson goes hand-in-hand with classroom material and they reinforce each other.
SCIENTIFIC TEACHING THEMES
ACTIVE LEARNING
- Students search for answers to questions prior to coming to lab. They are called upon in lab to share what they found.
- Student lab groups decide which promoter to build and formulate a testable hypothesis about the function of their promoter.
- Students use research literature and their understanding of how promoters work to choose which portion of a promoter to use.
- Students perform the laboratory procedures of cloning a promoter, transforming cells, and identifying colonies that contain the new promoter.
- Students with positively or negatively regulated promoters manipulate the growth conditions to test whether their promoter performs as predicted.
- Students interpret the quantitative data they produce.
- Students generate graphical representations of their data and conclusions.
- Students communicate their findings in oral and written presentations.
ASSESSMENT
- Students will assess whether their results support their hypothesis.
- Students present their results and interpretations in graded oral and written lab reports. Grading rubrics are available here: https://bio.davidson.edu/113/grade_Rubrics.html
- Students take pre- and post-surveys to measure comprehension of core concepts.
- Students submit their promoter designs and results to two online databases.
INCLUSIVE TEACHING
- Student lab groups at Davidson were assembled using CATME (http://info.catme.org (27) Team-maker to form lab groups with maximum diversity. Students used CATME weekly for peer- and self-evaluation of teamwork (see S1). CATME is a free web tool that helps students work together effectively as a team.
- Students must communicate, collaborate, and self-organize at many points throughout the laboratory sessions. Each person must contribute at multiple steps every week.
- For the graded oral presentations, students must be prepared to present any of the four parts of the presentation. They learn their assigned presentation part one hour before lab begins. Therefore, each student must master all fours sections of the report.
- Oral presentations include questions and answers where some students thrive more than others do.
- Students respond to feedback on oral presentation when preparing their written lab report in the form of a scientific paper.
LESSON PLAN
The Timeline for the pClone for Introductory Biology lab is available in supporting file S2.
INSTRUCTOR PREPARATION BEFORE CLASS
Prior to the first day of lab, students submit biographic and daily schedule information via CATME web tool (27). I employ the TEAM Maker function to produce diverse groups of four students each prior to their first lab (sixteen students per lab section). CATME TEAM Maker can also be used after the first lab for generation of diverse lab groups starting with the second lab meeting. Instructors can purchase the pClone Red plasmid from Carolina Biological starting in the fall of 2015. Once they have the plasmid, they can use the reagents supplied in the Carolina Biological kit, or assemble their own reagents and produce ample plasmid from a single miniprep. Details of the ligation preparation procedure are available in the kit or from the supporting file S11. We have found that students more fully understand GGA when they perform the paper activity during the GGA reaction. Instructors will want to print enough copies for each student to have one copy (see supporting file S13) as well as access to scissors and clear tape. We have provided several additional PowerPoint files that instructors can use to present and overview of GGA and experimental details. Instructors may use my online course site to see the materials employed before and during lab (https://bio.davidson.edu/113/113labscedule2015.html). Instructors will want to familiarize themselves with the synthetic biology registry of standardized DNA parts (http://parts.igem.org/Main_Page). All part numbers (such as J119137) are described in detail in the registry.
STUDENT PREPARATION FOR CLASS
Each week, students must complete homework questions that are relevant to that week's lab procedures (see supporting file S3). In the first minutes of lab, each group shares with the entire class what their answer to one of the pre-lab questions. Students learn sterile technique and how to plate transformed cells at the moment they perform these tasks when the instructor demonstrates once for them.
LAB SESSIONS
GENERAL INFORMATION
Because this is a four-week wet-lab module, we start slowly with dry lab activities to allow time in the classroom to cover DNA structure and gene regulation. Then, we cover how cloning works in general and GGA with pClone in particular (see S4). Students visit the two DNA registries they will be using so they realize their work will be part of a publicly accessible database of promoters and their functions. Knowing that their research will be part of a global research enterprise helps students recognize they are doing real science and not just reproducing outcomes that are already known.
Students take a pre-survey the first day of class to capture what they know prior to any instruction (see S5 and S6). There is no simple way into the literature for beginning students, so they are taught how to search for papers that describe the function of bacterial promoters and contain promoter DNA sequences. Students do not need to understand the entire paper and should focus on the function of the promoter in a prokaryote and whether it is positively or negatively regulated, or constitutive (always functions). From this starting point, they can develop a hypothesis about how their promoter design will function in E coli. To limit expense and increase cloning success rates, the total length of the experimental promoter cannot exceed 60 bp, which costs about $7.00 for each of the paired oligos. Our DNA provider, https://www.idtdna.com/, has a cost inflection point at 60 bases.
CHOOSING A PROMOTER - WEEK 1
Lab begins with a summary presentation to students about how pClone Red makes promoter research accessible (see S7). After question and answers, they search PubMed and Google Scholar to find open access research papers that characterize a promoter and give its complete sequence. We place few constraints on this assignment, other than the promoter must come from a prokaryote and it cannot be a well characterized promoters such as Plac, Ptet, Pbad, Ptac, etc. Students need to determine what cellular or environmental conditions influence the transcription of the gene. Reasonable perturbations include temperature, salts, and alternative carbon sources. Students should include the -10 and -35 regions in their designs and should note any protein-binding sites indicated for their promoter, though the binding sites do not have to be included in their promoters. Inclusion or exclusion of binding sites will influence the function of their promoter and should be considered when formulating their hypothesis. Students complete CATME self- and peer-evaluation (27).
DESIGNING THE PROMOTER - WEEK 2
The second lab begins with a presentation showing the details of how pClone works (see S8). After question and answers, students return to their papers and reevaluate their promoters. They can revise their hypotheses based on learning more in the lecture portion of the course or through independent study during the past week. The main point of this week is to generate the two oligonucleotides that will be used to form the double-stranded DNA (dsDNA) that will be base pair to form their promoter (see S9) and predetermined distinct sticky ends to facilitate directional cloning. Students use an online website called The Oligator (28) that generates the two oligonucleotide sequences and screens for internal BsaI restriction sites. Students will need to add the appropriate sticky end (as shown in Figure 1) to each oligonucleotide. Students must submit their promoter sequences to the Registry of Standard Parts (29). Students complete CATME self- and peer-evaluation.
CLONING THE PROMOTER - WEEK 3
The afternoon before lab, one student from each lab group comes to lab to mix their oligos, boil them for five minutes, and allow them to anneal overnight (see S10). During their regular lab meeting the next day, students dilute the cooled oligos further. After diluting the annealed oligos, students add them to the GGA mixture containing the receiving plasmid pClone Red, restriction enzyme BsaI, DNA ligase, ATP, and a buffer (see S11). The GGA mixture is prepared in advance by the instructor and stored in aliquots at -20C. GGA is placed in a thermocycler for 20-30 rounds of a two step cycle: 1 minute at 37C (optimal temperature for BsaI restriction enzyme) and 1 minute at 16C (optimal temperature for DNA ligase). Note, GGA is not PCR and there is no DNA polymerase. The temperature cycles cut and ligate the DNA multiple times to gradually remove the original promoter and ligate in the new promoter. More rounds produces higher success rates but take longer. The final step of 15 minutes of 37C reduces the number of undigested pClone Red plasmids. Students perform GGA with pClone Red and their experimental promoter as well as a negative control that contains all the same ingredients except it lacks their experimental promoter. The positive control plasmid containing a fixed promoter and RFP does not need to be subjected to GGA. Once GGA is completed, students transform three tubes of E. coli cells with three distinct DNA solutions: 1) negative control of pClone Red after GGA but no new promoter added; 2) positive control of RFP gene with Plac promoter (part number J04450); and 3) the experimental GGA reaction containing their new promoter (see S12). Each ligation is plated onto a separate LB amp plate and allowed to grow about 16 hours at 37C. While the GGA reaction is in the thermocycler, students perform the paper GGA exercise to better understand what is happening to pClone Red and their oligonucleotides (see S13). Students complete CATME self- and peer-evaluation.
CHARACTERIZING THE PROMOTER - WEEK 4
The afternoon prior to lab, one student from each group picks colonies and grows them overnight at 37C in 3mL of appropriate media sterile culture tubes (see S14). Students choose colonies after visualizing the transformed colonies on a UV light box: three green colonies from the negative control plate; three red colonies from the positive control plate; and three non-green colonies (red or colorless) from their experimental plate. No matter how few in number, red colonies indicate the promoter is working. If no red colonies appear on the experimental plate, then their experimental promoter is not strong or does not work at all under these growth conditions. For groups testing an induced or repressed promoter, they place their non-green experimental colonies in 3mL of LB amp alone as well as 3mL of LB amp plus any chemical or environmental conditions expected to regulate the promoter. Sterile toothpicks or yellow tips can be used to pick the colonies and transfer the cells to media. The same colony can be transferred to two tubes of media by a single toothpick or yellow tip. Constitutive promoters do not require additional growth conditions.
When lab starts, students photograph the colonies on plates and culture tubes of overnight cells. Colonies fluoresce best on UV light if the lids are removed and plates are placed colony side down. Be sure to use protective shielding when working with UV light. Alternatively, darker red colonies can be photographed under white light if placed on white paper and the lids are removed. Tubes over overnight cultures are best photographed under white light with white paper placed behind the tubes. Photographs should be taken before placing aliquots of the overnight cultures in a 96-well plate reader to measure RFP fluorescence (585 nm excitation and 615 nm emission) to measure expression from the experimental and control promoters and light absorbance (OD590) to quantify cell density. Students calculate the fluorescence to absorbance ratio to normalize the amount of RFP signal to the number of cells present (see Options to Extend the lesson to measure promoter strength in lieu of using a fluorometer). Fluorescence and absorbance do not have absolute units so they are measured in arbitrary units. Students spend their remaining time interpreting their results and generating graphical representations of their data. They also submit their results to the two DNA registries, including fold induction when comparing the Plac promoter to their experimental promoter (Figure 3). Students submit all their information to the Registry of Functional Promoters (30). Students complete CATME self- and peer-evaluation.
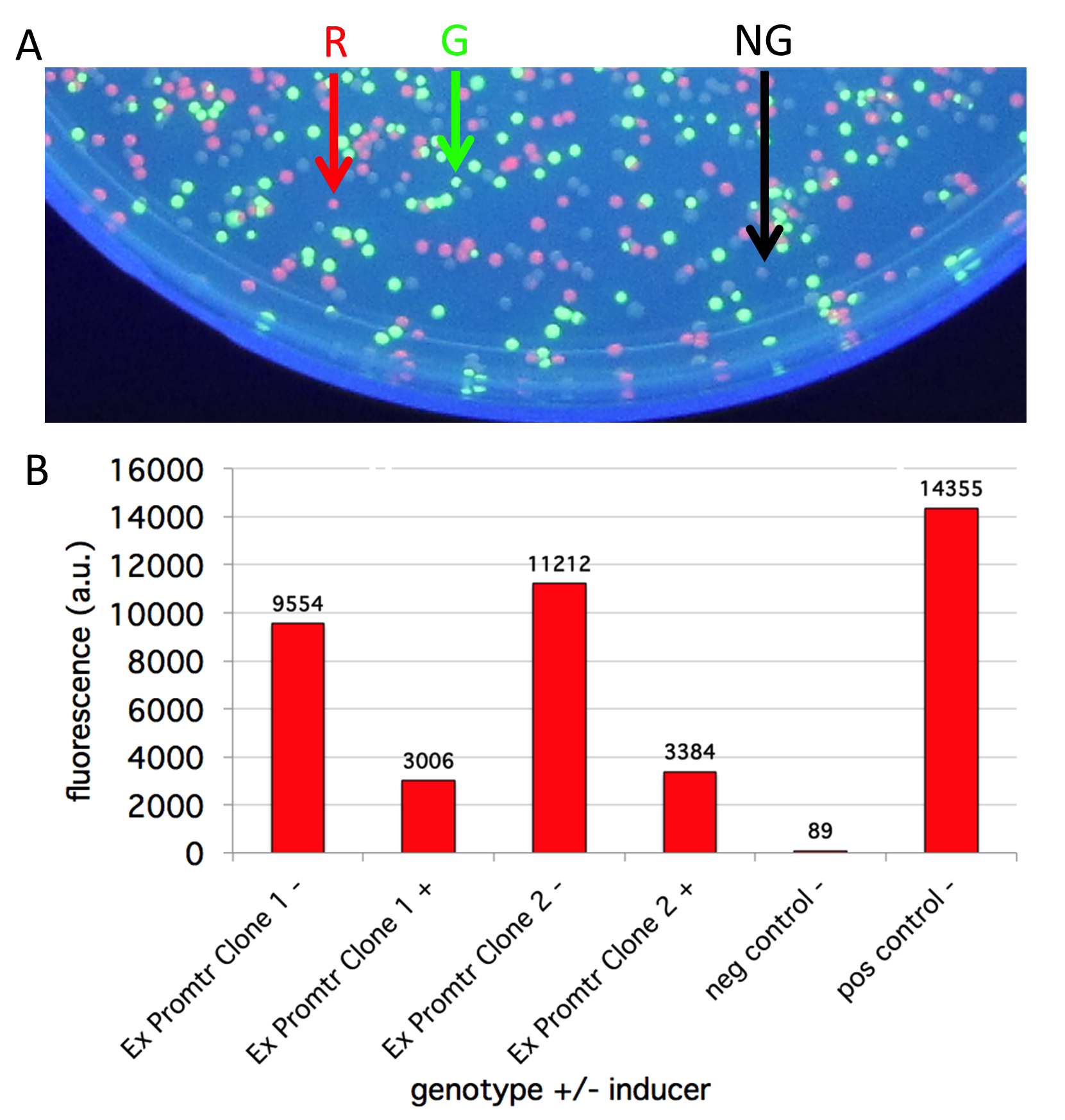
Figure 3. Student data with functional, experimental promoter. A) Portion of experimental ligation and transformation plate showing three colors of colonies as indicated by colored arrows. B) Graph showing student data from a promoter that they hypothesized would be inducible (+) but proved to be repressed by their predicted inducer. When the inducer was omitted (-), the colonies fluoresced about 70% as bright as the positive control (J04450). Negative control colonies (J100091) shows only background level fluorescence. (R = red, G = green, NG = not green).
PRESENTING THE RESULTS - WEEKS 5 AND 6
Outside of lab, students prepare and practice their oral presentations. Each presentation is limited to fifteen minutes and divided into four sections - introduction, methods, results, and discussion. Each student is assigned to a different section one hour before lab begins so that every student must prepare all four sections instead of just the one section he or she ends up presenting. Students receive anonymous written feedback from their peers and graded evaluation from the instructor (see S15). Students incorporate this feedback when they write their lab report, which they submit the following week. Oral and written research presentations are graded and returned to students so they can benefit from my feedback. Students complete CATME self- and peer-evaluation.
OPTIONS TO EXTEND THE LESSON
The pClone lesson has four possible extensions, all of which are appropriate for introductory biology students.
EXTENSION 1: QUANTIFYING WITHOUT A FLUOROMETER.
If a fluorometer is not available, students can photograph colonies and then use the free software ImageJ on a computer to quantify the redness of the colonies (31). This photographic method is not as accurate as using a fluorometer, but it will give quantitative data that students can use to assess relative promoter function. The details for using ImageJ are in the supplemental materials (see S16; (24). It is worth noting that unlike GFP, RFP is easily visualized with normal room lighting.
EXTENSION 2: QUANTIFYING WITHOUT A UV LIGHT BOX.
If you do not have a UV light box, or want to use a different reporter, you can use pClone Blue (part J119313) that has amilCP Blue as the reporter gene instead of RFP (32). pClone Blue is paired with a positive control plasmid (part J119128) that contains the well-characterized promoter P5. As with pClone Red, amilCP Blue can be quantified by photographs of colonies taken in visible light and analyzed by ImageJ. Alternatively, you can grow the positive control blue cells and produce a serial dilution. From this dilution series, you can measure absorbance at 400 nm and generate a standard curve against which you can compare any experimental promoters (see S17).
EXTENSION 3: DISSECT FUNCTION OF BASES IN KNOWN PROMOTER.
Rather than finding a new promoter from the literature, students can mutate a well-characterized promoter such as Plac, Ptac, P5, etc. This extension is described in detail in the companion CourseSource Genetics Lesson by Eckdahl and Campbell. Mutational analysis of a promoter could be combined with the current lesson by having student introduce mutations into promoters that are expected to be under positive or negative control.
EXTENSION 4: VERIFY CLONING OF NON-FUNCTIONAL PROMOTER.
One limitation of pClone results is that sometimes plasmids contain no promoter at all, because the original promoter was removed and the experimental promoter was not ligated into the receiving plasmid. The result are colorless colonies, which is same phenotype as a plasmid with an experimental promoter that does not work or is very weak. To distinguish these two possible genotypes, you could either have some plasmids sequenced, or you could use PCR to distinguish the absence of the 70 bp PlacIQ1 promoter in the original plasmid from an inserted <=60bp experimental promoter (see S18). I have used PCR screening with my introductory students before, but dropped this step because it was not central to my learning goals.
TEACHING DISCUSSION
TECHNICAL ISSUES
Perhaps the most compelling aspects of the pClone Red module is that it has worked for 100% of all lab groups composed of first term, first year students. Further, pClone Red is inexpensive and does not require any specialized equipment unless you want to quantify the RFP by fluorescence. Students have cloned many different promoters and about a third of them have worked as predicted. Cloning a non-functional promoter is a good lesson for students to realize that their hypotheses can be wrong even when they did everything correctly. Unexpected results send students back to the literature where they are looking for good explanations for their results (Figure 3B). Typically, students have truncated the promoter too much, but that is a fine outcome and consistent with the learning goals and objectives.
STUDENT COMMENTS
Students often start this lab lesson with a fair bit of confusion about what they are doing, but early confusion is not always bad. As they progress into the semester, they learn more and more about promoters so their lab experiences make more sense with time. Students have repeatedly told me in person and indicated on anonymous course evaluations that they like doing real promoter research rather than canned labs. These comments are consistent with the data discussed in Vision and Change (1) as well as BIO2010 (4). Cognitive psychologists have documented that students learn best when they construct their own knowledge. This construction process is consistent with students moving from initial confusion to eventual clarity about how a promoter works and how their research with pClone Red tested the function of their promoter (33). As an alternative, students could mutate a known promoter, as described in the companion Genetics Lesson by Eckdahl and Campbell.
LEARNING OUTCOMES
Recently, a lot of attention has been given to Classroom-based Undergraduate Research Experiences (CURE; (22,23,25,26,9,34-39). The pClone Red promoter-research lesson provides an easy way to provide scale up CURE opportunities in all teaching lab sections. In our paper describing pClone (24), we showed that students accomplished six of the nine learning objectives and are listed here. My students could not design a PCR experiment to verify a promoter was cloned because this became an extension I no longer included. Students did not know how to design an experiment to test promoter strength even though they had done exactly that in lab. Perhaps the wording of the question was unclear, but further investigation is warranted on this objective. Surprisingly, they did not improve their ability to define a type IIs restriction enzyme, which is a low-level cognitive function on Bloom's taxonomy (40), but this point was not emphasized because it was minor compared to other goals and objectives.
The pClone Red Introductory Biology lesson addresses one of the core concepts and several core competencies outlined in Vision and Change (1). It provides faculty with a low cost lab module that has allowed 100% of the student lab groups to clone a 60bp promoter they designed. The students evaluated their hypotheses about how their promoters would function. Students are actively engaged in constructing their own understanding about promoters while using many learning strategies. Feedback on course evaluations has been very favorable and students synthesize their knowledge in oral and written laboratory reports that confirm that many of the learning goals and objectives are accomplished.
A common misconception is that different cell types in a multicellular organism contain different genes rather the correct understanding that all cells containing the same genes that are differentially regulated at the transcriptional level. Working with promoters over an extended period and testing the strength of a promoter they designed helps students gain a greater appreciation for the central role promoters play in cell and molecular biology. Furthermore, students learn to clone using a new method that is substantially easier and more effective than the traditional approach that often requires gel purification and the need for sticky ends generated when type II restriction enzymes cut within their recognition sites. Students often think that the newest method is normal, rather than being as impressed as their instructors are about the improvements. Despite their inability to appreciate the advantages of the new methods, it is very common for students to share their excitement during lab that this synthetic biology promoter lab module is real research that other biologists can access and use in their own research. It should not be surprising that students prefer to do meaningful lab work, rather than busywork replicating previous results, or studying a new question that is of little interest by the scientific community. It is exciting to offer first year students a meaningful research experience that is inexpensive and allows every lab group to clone and test a new promoter sequence. For four years, 100% of the student lab groups have successfully cloned their designed promoter, though only about 25% perform as expected which produces a very rich learning environment when students can learn that unexpected results are normal in research.
This lesson spells out the details of how to implement a course-based, undergraduate research experience (CURE) within the budget and time constraints of a typical lab. In particular, the degree of difficulty and cost of the lab are consistent with a typical introductory biology course intended for majors and potential majors. In 2014, we published a detailed description of the learning objectives and student assessment data in CBE - Life Sciences Education (24).
SUPPORTING MATERIALS
- S1. pClone Intro-Links to CATME and How to Pipet
- S2. pClone Intro-Timeline of pClone Red Introductory Biology Laboratory Lesson
- S3. pClone Intro-Weekly Questions for Students to Answer Prior to Lab
- S4. pClone Intro-Links to Introduction to Synthetic Biology and Cloning
- S5. pClone Intro-Pre- and Post-Assessment Questions, no answers
- S6. pClone Intro-Pre- and Post-Assessment Questions, with answers
- S7. pClone Intro-Overview of Golden Gate Assembly for Promoter Research
- S8. pClone Intro-Details of Golden Gate Assembly for Promoter Research
- S9. pClone Intro-Designing Experimental Promoter
- S10. pClone Intro-Annealing Promoter Oligonucleotides
- S11. pClone Intro-Golden Gate Assembly with pClone Red
- S12. pClone Intro-Transforming DNA into E. coli Cells
- S13. pClone Intro-Paper Activity for GGA Cloning into pClone
- S14. pClone Intro-Phenotype Analysis after Transformation
- S15. pClone Intro-Lighthearted BINGO Scorecard to Improve Oral Presentations
- S16. pClone Intro-Quantifying pClone Red Promoter Strength with ImageJ (no fluorometer)
- S17. pClone Intro-Using pClone Blue instead of pClone Red (no UV light
- S18. pClone Intro-pClone Genotyping to Verify Promoter Cloning
ACKNOWLEDGEMENTS
We thank Brian Cronk, Corinne Andresen, Paul Frederick, Samantha Huckuntod, Claire Shinneman, Annie Wacker, and Jason Yuan for their help in developing pClone Red. Support was provided by the National Science Foundation RUI grants MCB-1120578 to Davidson College and MCB-1120558 to MWSU; Howard Hughes Medical Institute grant 52006292 to Davidson College; the James G. Martin Genomics Program at Davidson College; and the MWSU Program of Research, Teaching, and Applied Learning (PORTAL). A.M.C. and T. E. are members of GCAT SynBio. pClone Red is being marketed by Carolina Biological and therefore the authors have a conflict of interest to declare.
References
- AAAS. 2011. Vision and Change: A Call to Action, Final Report. http://visionandchange.org/finalreport/ Accessed November 13.
- Barsoum MJ, Sellers PJ, Campbell AM, Heyer LJ, Paradise CJ. 2013. Implementing recommendations for introductory biology by writing a new textbook. CBE Life Sci Educ 12:106-116.
- Eckdahl T, Campbell AM. 2015. CourseSource pClone Genetics Lesson.
- Council NR. 2000. BIO 2010: transforming undergraduate education for future research biologists. http://www.nap.edu/catalog.php?record_id=10497. Accessed November 13.
- Seymour EHA, Laursen SL, DeAntoni T. 2004. Establishing the benefits of undergraduate research for undergraduates in the sciences: first findings from a three-year study. Sci Educ 88:493-594.
- Lopatto D. 2006. Undergraduate research as a catalyst for liberal learning. Peer Rev 8:22-25.
- Laursen S, Hunter A, Seymour E, Thiry H. 2010. Undergraduate Research in the Sciences: Engaging Students in Real Science. Real Science San Francisco, Jossey-Bass:320.
- Slater SJ, Slater TF, Bailey, M. J. 2010. Discipline-Based Education Research: A scientist's Guide, New York, New York.
- Harrison M, Dunbar D, Ratmansky L, Boyd K, Lopatto D. 2011. Classroom-Based Science Research at the Introductory Level: Changes in Career Choices and Attitude. CBE--Life Sciences Education 10:279-286.
- Haynes KA, Broderick ML, Brown AD, Butner TL, Dickson JO, Harden WL, Heard LH, Jessen EL, Malloy KJ, Ogden BJ, Rosemond S, Simpson S, Zwack E, Campbell AM, Eckdahl TT, Heyer LJ, Poet JL. 2008. Engineering bacteria to solve the burnt pancake problem. J Biol Eng 2:8.
- Baumgardner J, Acker K, Adefuye O, Crowley ST, DeLoache W, Dickson JO, Heard L, Martens AT, Morton N, Ritter M, Shoecraft A, Treece J, Unzicker M, Valencia A, Waters M, Campbell AM, Heyer LJ, Poet JL, Eckdahl TT. 2009. Solving a Hamiltonian Path Problem with a Bacterial Computer. J Biol Eng 3:11.
- Eckdahl TT, Campbell AM, Heyer LJ, Poet JL. 2010. Synthetic Biology as a new opportunity for multidisciplinary undergraduate research. CUR Quarterly 30:42-48.
- Wolyniak MJ, Alvarez CJ, Chandrasekaran V, M. GT, Holgada A, J. JC, W. MR, L. PA, J. S, M. WT, Y. Y. 2010. Building Better Scientists through Cross-Disciplinary Collaboration in Synthetic Biology: A Report from the Genome Consortium for Active Teaching Workshop 2010. CBE Life Sci Educ 9:399-404.
- Pearson B, K. H. Lau, A. Allen, J. Barron, R. Cool, K. Davis, W. DeLoache, E. Feeney, A. Gordon, J. Igo, A. Lewis, K. Muscalino, M. Parra, P. Penumetcha, V. G. Rinker, K. Roland, X. Zhu, J. L. Poet, T. T. Eckdahl, L. J. Heyer and A. M. Campbell. 2011. Bacterial Hash Function Using DNA-Based XOR Logic Reveals Unexpected Behavior of the LuxR Promoter Interdisciplinary BioCentral 3:10.
- Sawyer EM, C. Barta, R. Clemente, M. Conn, C. Davis, C. Doyle, M. Gearing, O. Ho-Shing, A. Mooney, J. Morton, S. Punjabi, A. Schnoor, S. Sun, S. Suresh, B. Szczepanik, D. L. Taylor, A. Temmink, W. Vernon, A. M. Campbell, L. J. Heyer, J. L. Poet and T. T. Eckdahl 2012. Bacterial Logic Devices Reveal Unexpected Behavior of Frameshift Suppressor tRNAs. Interdisciplinary BioCentral 4:10.
- Eckdahl TT, Campbell AM, Heyer LJ, Poet JL, Blauch DN, Snyder NL, Atchley DT, Baker EJ, Brown M, Brunner EC, Callen SA, Campbell JS, Carr CJ, Carr DR, Chadinha SA, Chester GI, Chester J, Clarkson BR, Cochran KE, Doherty SE, Doyle C, Dwyer S, Edlin LM, Evans RA, Fluharty T, Frederick J, Galeota-Sprung J, Gammon BL, Grieshaber B, Gronniger J, Gutteridge K, Henningsen J, Isom B, Itell HL, Keffeler EC, Lantz AJ, Lim JN, McGuire EP, Moore AK, Morton J, Nakano M, Pearson SA, Perkins V, Parrish P, Pierson CE, Polpityaarachchige S, Quaney MJ, Slattery A, Smith KE, Spell J, et al. 2015. Programmed evolution for optimization of orthogonal metabolic output in bacteria. PLoS One 10:e0118322.
- Biotechnology and Biological Sciences Research Council DSaTL, Engineering and Physical Science Research Council, Medical Research Council 2011. Joint Synthetic Biology Initiative (JSBI). http://www.bbsrc.ac.uk/funding/grants/priorities/synthetic-bio/. Accessed November 13.
- Knight TF. 2003. Idempotent Vector Design for Standard Assembly of Biobricks. MIT Synthetic Biology Working Group.
- Campbell AM. 2005. Meeting report: synthetic biology Jamboree for undergraduates. Cell Biol Educ 4:19-23.
- Khalil AS, Collins JJ. 2010. Synthetic biology: applications come of age. Nat Rev Genet 11:367-379.
- Kahl LJ, Endy D. 2013. A survey of enabling technologies in synthetic biology. J Biol Eng 7:13.
- Wood WB, Mathematics NRCCoPfASo, Science in American High S. 2002. Advanced high school biology in an era of rapid change: a summary of the biology panel report from the NRC Committee on Programs for Advanced Study of Mathematics and Science in American High Schools. Cell Biol Educ 1:123-127.
- CollegeBoard. 2011. AP Biology Curriculum Framework 2012-2013. New York.
- Campbell AM, Todd Eckdahl, Brian Cronk, Corinne Andresen, Paul Frederick, Samantha Huckuntod, Claire Shinneman, Annie Wacker, and Jason Yuan. 2014. pClone: Synthetic Biology Tool Makes Promoter Research Accessible to Beginning Biology Students. CBE Life Sciences Education 13:285-296.
- Weber E, Gruetzner R, Werner S, Engler C, Marillonnet S. 2011. Assembly of designer TAL effectors by Golden Gate cloning. PLoS One 6:e19722.
- Werner S, C. Engler, E. Weber, R. Gruetzner, Marillonnet, S. 2012. Fast track assembly of multigene constructs using Golden Gate cloning and the MoClo system. Bioeng Bugs 3:38-43.
- 2003 Co. 2003. CATME Team-maker. http://info.catme.org. Accessed March 11.
- Meador S, Streb, S., Harden, L., Whitesides, T., Heyer, L. J. . 2010. The Oligator http://gcat.davidson.edu/igem10/ Accessed March 11.
- Group MW. 2013. Registry of Standard Biological Parts http://partsregistry.org/Main_Page Accessed March 11.
- Campbell AM, Hatfield, B., Heyer, L.J., Eckdahl, T.T. . 2013. Registry of Functional Promoters. http://gcat.davidson.edu/RFP/ Accessed March 11.
- Rasband WS. 1997-2012. ImageJ, U. S. National Institutes of Health, Bethesda, Maryland, USA. http://imagej.nih.gov/ij/. Accessed March 11.
- iGEM TU-S. 2011. amilCP, blue chromoprotein. http://parts.igem.org/Part:BBa_K592009 Accessed November 13.
- Council NR. 2000. How People Learn: Brain, Mind, Experience, and School: Expanded Edition. National Academies Press, Washington, DC.
- Lopatto D. 2007. Undergraduate research experiences support science career decisions and active learning. CBE Life Sci Educ 6:297-306.
- Wei CA, Woodin T. 2011. Undergraduate research experiences in biology: alternatives to the apprenticeship model. CBE Life Sci Educ 10:123-131.
- David Lopatto CH, Christopher J. Jones, Don Paetkau, Vidya Chandrasekaran, David Dunbar, Christy MacKinnon, Joyce Stamm, Consuelo Alvarez, Daron Barnard, James E. J. Bedard, April E. Bednarski, Satish Bhalla, John M Braverman, Martin Burg, Hui-Min Chung, Randall J. DeJong, Justin R. DiAngelo, Chunguang Du, Todd T. Eckdahl, Julia Emerson, Amy Frary, Donald Frohlich, Anya L. Goodman, Yuying Gosser, Shubha Govind, Adam Haberman, Amy T. Hark, Arlene Hoogewerf, Diana Johnson, Lisa Kadlec, Marian Kaehler, S. Catherine Silver Key, Nighat Kokan, Olga R. Kopp, Gary A. Kuleck, Jane Lopilato, Juan C. Martinez-Cruzado, Gerard McNeil, Stephanie Mel, Alexis Nagengast, Paul J. Overvoorde, Susan Parrish, Mary Preuss, Laura D. Reed, E. Gloria Regisford, Dennis Revie, Srebrenka Robic, Jennifer A. Roecklien-Canfield, Anne G. Rosenwald, Michael R. Rubin, Kenneth Saville, Stephanie Schroeder, Karim Sharif, Mary Shaw, Gary Skuse, Christopher D Smith, Mary Smith, Sheryl T. Smith, Eric P. Spana, Mary Spratt, Aparna Sreenivasan, Jeffrey S. Thompson, Matthew Wawersik, Michael Wolyniak, James Youngblom, Leming Zhou, Jeremy Buhler, Elaine Mardis, Wilson Leung, Christopher D. Shaffer, Jennifer Threlfall, Sarah C. R. Elgin. 2014. A Central Support System Can Facilitate Implementation and Sustainability of a Classroom-Based Undergraduate Research Experience (CURE) in Genomics. CBE Life Sci Educ 13:711-723.
- Hanauer, DI and Dolan EL. 2014. The Project Ownership Survey: Measuring Differences in Scientific Inquiry Experiences. CBE Life Sci Educ 13:149-158.
- Corwin LA, Graham MJ, Dolan EL. 2015. Modeling Course-Based Undergraduate Research Experiences: An Agenda for Future Research and Evaluation. CBE Life Sci Educ 14:1-13.
- Russell JE, D'Costa AR, Runck C, Barnes DW, Barrera AL, Hurst-Kennedy J, Sudduth EB, Quinlan EL, Schlueter M. 2015. Bridging the Undergraduate Curriculum Using an Integrated Course-Embedded Undergraduate Research Experience (ICURE). CBE Life Sci Educ 14.
- Bloom BS. 1956. Taxonomy of Educational Objectives, Handbook I: The Cognitive Domain, New York.
Article Files
Login to access supporting documents
Using Synthetic Biology and pClone Red for Authentic Research on Promoter Function: Introductory Biology (identifying new promot(PDF | 837 KB)
S1. pClone Intro-Links to CATME and How to Pipet.pptx(PPTX | 523 KB)
S2. pClone Intro-Timeline of pClone Red Introductory Biology Laboratory Lesson.pdf(PDF | 41 KB)
S3. pClone Intro-Weekly Questions for Students to Answer Prior to Lab.docx(DOCX | 64 KB)
S4. pClone Intro-Links to Introduction to Synthetic Biology and Cloning.pptx(PPTX | 1 MB)
S5. pClone Intro-Pre- and Post-Assessment Questions no answers .docx(DOCX | 23 KB)
S6. pClone Intro-Pre- and Post-Assessment Questions with answers .docx(DOCX | 48 KB)
S7. pClone Intro-Overview of Golden Gate Assembly for Promoter Research.pptx(PPTX | 750 KB)
S8. pClone Intro-Details of Golden Gate Assembly for Promoter Research.pptx(PPTX | 5 MB)
S9. pClone Intro-Designing Experimental Promoter.docx(DOCX | 179 KB)
S10. pClone Intro-Annealing Promoter Oligonucleotides.docx(DOCX | 23 KB)
S11. pClone Intro-Golden Gate Assembly with pClone Red.docx(DOCX | 26 KB)
S12. pClone Intro-Transforming DNA into E. coli Cells.docx(DOCX | 24 KB)
S13. pClone Intro-Paper Activity for GGA Cloning into pClone.pptx(PPTX | 68 KB)
S14. pClone Intro-Phenotype Analysis after Transformation.docx(DOCX | 627 KB)
S15. pClone Intro-Lighthearted BINGO Scorecard to Improve Oral Presentations.pptx(PPTX | 55 KB)
S16. pClone Intro-Quantifying pClone Red Promoter Strength with ImageJ no fluorometer.docx(DOCX | 143 KB)
S17. pClone Intro-Using pClone Blue instead of pClone Red no UV light.docx(DOCX | 793 KB)
S18. pClone Intro-pClone Genotyping to Verify Promoter Cloning.docx(DOCX | 304 KB)
- License terms
Comments
Comments
There are no comments on this resource.