Serotonin in the Pocket: Non-covalent interactions and neurotransmitter binding
Published online:
Abstract
This lesson introduces beginning biochemistry students to the concept of a biomolecule binding site, such as a neurotransmitter receptor site or an enzyme active site. A simple organic molecule, the neurotransmitter serotonin, is the focus of the lesson. A pre-activity guides students in their review of non-covalent interactions prior to engaging in the lesson. During the lesson, students are asked to consider three different points on the serotonin molecule where non-covalent intermolecular forces may act to hold the serotonin molecule in a binding site. After students decide on the appropriate non-covalent forces, they are presented with variations of the serotonin structure. In each case, students are asked to determine whether binding is enhanced or weakened in light of the change. Finally, students explore the likelihood that other common metabolites will also bind in the serotonin-binding site. After completing this lesson, students are able to explain the role of noncovalent interactions in the binding of small metabolite molecules to biologically relevant binding pockets such as receptor binding sites or enzyme active sites.
Citation
Werth, M.T. 2017. Serotonin in the Pocket: Non-covalent interactions and neurotransmitter binding. CourseSource. https://doi.org/10.24918/cs.2017.14Society Learning Goals
Biochemistry and Molecular Biology
- Macromolecular Structure Determines Function and Regulation
- What factors determine structure?
- How is structure (and hence function) of macromolecules governed by foundational principles of chemistry and physics?
Lesson Learning Goals
- Students will understand the role of non-covalent interactions in the process of a metabolite (serotonin) binding to a protein-binding site.
- Students will improve their knowledge of the characteristics of non-covalent forces.
- Students will improve their understanding of the relationship between distance and the strength of a non-covalent interaction.
Lesson Learning Objectives
- Students will design a binding site for the neurotransmitter serotonin.
- Students will be able to determine the effect of a change in molecular orientation on the affinity of the molecule for the binding site.
- Students will be able to determine the effect of a change in molecular charge on the affinity of the molecule for the binding site.
- Students will be able to better differentiate between hydrogen bond donors and acceptors.
- Students can use this knowledge to design binding sites for other metabolites.
Article Context
Course
Article Type
Course Level
Bloom's Cognitive Level
Vision and Change Core Concepts
Class Type
Class Size
Audience
Lesson Length
Pedagogical Approaches
Principles of How People Learn
Assessment Type
INTRODUCTION
This lesson was created to introduce beginning biochemistry students to the role of noncovalent interactions during biochemical binding processes. Loertscher and coauthors (1,2) have identified the “physical basis of interactions” as a threshold concept in biochemistry. Wright and coauthors (3) describe the “hydrophobic effect” and “hydrogen bonds and other noncovalent interactions” as important concepts from chemistry for the education of undergraduate biochemistry students. This sentiment is echoed by Tansey and coauthors (4), who add that the “structural organization of interacting chemical groups in a binding site or an active site lends a high degree of specificity to these interactions.” The binding pocket concept reappears frequently during study of biochemistry. Presenting non-covalent forces and the binding pocket at the beginning of the semester, allows one to have a richer discussion of many topics throughout the semester. For example, I have students read Watson and Crick’s original paper on the discovery of DNA. Their paper is filled with examples of hydrogen bonding and other non-covalent interactions.
Non-covalent interactions are taught in both beginning biology and chemistry courses at my institution (5,6). However, it became apparent to me that biochemistry students need additional work with these concepts. There are multiple reasons for this difficult transition. One is simply context. Biochemists rarely, if ever, refer to melting points or boiling points. Another issue is the multitude of variations in which the electrostatic interaction appears. Students are only beginning understand that a hydrogen bond and pi-cation interaction are simply variations of an electrostatic interaction. While general chemistry textbooks (6) provide an introduction to these non-covalent interactions, a paper by Bissantz, Kuhn, and Stahl presents a more biochemically relevant perspective (7). Finally, there is hydrophobic effect. Beginning biochemistry students have a vague understanding at best of the hydrophobic effect. A typical discussion of the hydrophobic effect describes an entropic effect involving solvent water molecules (8). However, as a recent report suggests, we still don’t have a thorough understanding of the origin of this important effect (9). Regardless, the hydrophobic effect appears biochemistry and students need a beginning knowledge of this effect to progress in biochemistry.
Currently, the Protein Data Bank provides PoseView images for over 90% of the ligand-biomolecule complexes found in the data bank (10,11). PoseView images provide two-dimensional representations of the essential characteristics of a binding site. However, as for most visual representations, the information presented in these images is not obvious to a beginning biochemistry student. This lesson introduces students to the information illustrated by these PoseView images.
In fall 2008, Tian and coworkers (12) presented a binding site image showing that the inhibitor they had designed for the enzyme o-succinylbenzoyl-CoA synthetase bound to two adjacent binding sites, an OSB-site and an ATP site. I adapted that image for a final exam question (see 2008 final exam in S1). The question asked the students to suggest amino acids that would used to bind the inhibitor at specific points. This question was presented to students on the “open-book” portion of the final exam. The question worked well and I wrote another question for the final exam in 2009. I then realized that the binding pocket diagram lent itself to development of an in-class lesson. Classroom work with binding pocket diagrams made it possible to move these questions to the closed book portion of the exams.
Since fall 2010, binding site structure is the first topic I teach in my biochemistry course. The intent is to demonstrate the applicability of previously learned knowledge about intermolecular forces (e.g. hydrogen bonding) to understand binding interactions in the context of biochemical events. Developing the concept of binding at the beginning of the course accomplishes several things. First, the threshold concept for the physical basis of interactions is addressed at the outset (1,2). When students suggest a negative charge opposite the positively charged protonated primary amine in the serotonin molecule, there is some question as to what structure might provide a negative charge. This question leads to discussion of the twenty amino acids as possible sources for the various interactions that make the serotonin-binding pocket. Because negative charges in biochemistry usually come from the conjugate bases of weak acids, a discussion of pH effects becomes appropriate. For most students, this lesson provides their first introduction to the hydrophobic effect. Students struggle with the concept at this point. However, introduction here creates the opportunity to revisit the “hydrophobic effect” when we cover lipids and membranes. Likewise, we revisit hydrogen bonding during discussion of carbohydrate and nucleic acid structures.
This lesson uses a guided-inquiry approach during which students typically work in groups of three or four. I have prepared a pre-activity assignment that guides students in their review of non-covalent intermolecular forces. At the beginning of the classroom lesson, the students briefly review their responses to the pre-activity. The lesson then begins with the chemical structure of the neurotransmitter serotonin placed in a binding pocket diagram (Figure 1).

Figure 1. A binding pocket diagram is shown where students are to select the appropriate non-covalent interactions at positions A, B and C to create a binding site that would be specific to the serotonin neurotransmitter molecule.
In the example shown, the binding pocket diagram has three positions where the binding site interacts with the serotonin molecule. Students are asked to select the most appropriate binding force at each position. Besides the obvious student interest in a neurotransmitter molecule, serotonin was chosen because it provides examples of hydrogen bonding, ionic interactions and hydrophobic interactions in a biochemical binding site. After the students decide on the most appropriate force at each of the three positions, they are instructed to keep these choices fixed for the remainder of the lesson. The lesson then proceeds to alter the initial structure of the serotonin molecule. In one case, a simple carbon-carbon single bond rotation moves the protonated primary amino group to a new position in the binding diagram. Students are asked to determine whether that change increases, decreases, or has no effect on the overall binding of the serotonin molecule to the receptor binding site. The other two variations involve changing the protonation state of the primary amino group and flipping the orientation of the serotonin molecule in the binding pocket. The last part of the lesson explores the question of other metabolites (e.g. dopamine, glucose, and tryptophan) binding in the serotonin-binding site that the students have created.
INTENDED AUDIENCE
This lesson is intended for a first semester upper–level undergraduate biochemistry students. The lesson was developed for a classroom of mostly junior-level biology, biochemistry, and chemistry majors at a small liberal arts college.
REQUIRED LEARNING TIME
A short pre-activity assignment is assigned prior to the day of in-class work. Student groups can complete the in-class lesson in a single 50-minute class session. A more detailed timeline with typical class progress through the lesson is provided in Table 1.
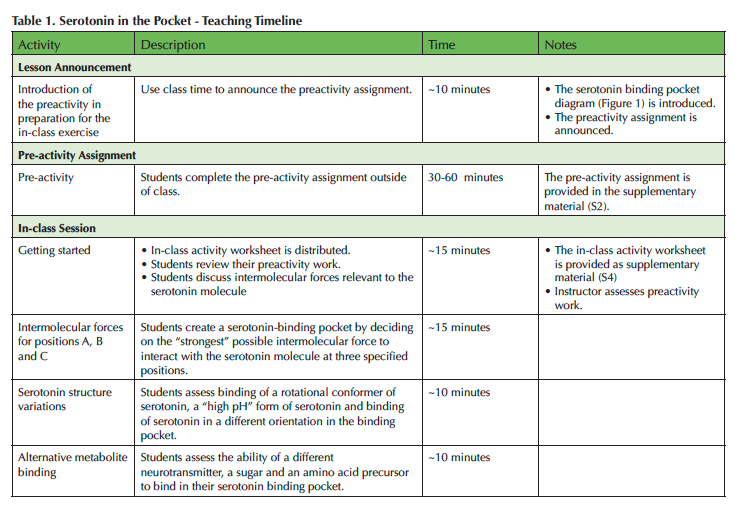
Table 1. Serotonin in the pocket - Teaching Timeline
PRE-REQUISITE STUDENT KNOWLEDGE
Students should have prior knowledge of hydrogen bonding, ionic interactions, and van der Waal’s forces (a.k.a. London dispersion forces.) Knowledge of the hydrophobic effect is often lacking in beginning biochemistry students and the “pre-activity assignment” seeks to introduce that topic. Noncovalent interactions are discussed in most general and organic chemistry courses. Students should be familiar with chemical structures representing organic molecules from a first-semester organic chemistry course.
PRE-REQUISITE TEACHER KNOWLEDGE
Instructors should be well versed in the characteristics of non-covalent intermolecular forces. The relevant non-covalent interactions are hydrogen bonding, ionic interactions, London dispersion forces, and the hydrophobic effect. A biochemistry textbook should provide a starting point for review and the article by Bissantz and coauthors (7) is another resource. Instructors should understand the relative strengths, distance dependencies, and the physical basis that underlies each of these forces. In addition, instructors should understand the effect that a pH change may have on these interactions.
SCIENTIFIC TEACHING THEMES
ACTIVE LEARNING
Outside of class, students are expected to prepare by reviewing the characteristics and properties of the non-covalent interactions learned in previous courses.
During class, students explore their understanding of the non-covalent interactions during their review of the pre-activity work. During parts B and C of the lesson, students apply their knowledge of non-covalent interactions to the molecule serotonin. Next, the groups actively decide which non-covalent forces in positions A, B, and C will give them the best serotonin binding site. The remaining portions of the lesson challenge students to assess the binding of different forms of serotonin and other metabolite molecules to their serotonin binding pocket.
ASSESSMENT
During the lesson, the student responses to the questions and student questions to the instructor provide feedback as to their progress. The summative assessment of this lesson consists of exam questions (S1) that use the binding pocket diagram. A new molecule is chosen to replace the serotonin and students are asked to suggest the forces used at specific locations. In the case of the author’s classes, the students have learned the amino acid structures for the same exam. This expectation allows me to ask students to identify possible amino acids that could provide the intended binding force (e.g., an ionic bond.) In addition, I also use multiple-choice questions to assess student’s knowledge of the intermolecular forces in general.
INCLUSIVE TEACHING
Since this lesson reviews knowledge of noncovalent forces from previous courses, it is common that different students will remember this background knowledge differently. Therefore, each student has the potential to contribute to the group work. The students are instructed to create a binding site by choosing the appropriate interaction at each position. Again, each student has the potential to contribute to the group effort. Because the chosen binding site is the basis for the remainder of the lesson, the team is motivated to consider all possibilities to ensure a successful outcome.
The lesson includes interpretation of both text and diagrams creating a need for different learning modalities.
LESSON PLAN
I introduce the lesson on the first day of class with a brief introductory lecture (about five to ten minutes) that provides an overview of the lesson. Students are told that the lesson is intended to introduce the concept of binding using the binding of the neurotransmitter serotonin to its receptor as an example. As part of the introduction, students are shown the binding pocket diagram with the serotonin molecule in position (Figure 1). Students are told that an intermolecular interaction will be required at each position, denoted A, B and C. It will be their task to determine which non-covalent interaction is most appropriate at each position. The pre-activity (S2) is assigned for the next class period. An answer key for the pre-activity is provided (S3). The next class period is devoted to the in-class group lesson.
The pre-activity introduces the purpose of the lesson. Students are then asked to provide short written descriptions of several intermolecular forces. Most students have already encountered London dispersion forces, dipole-dipole forces, ionic interactions, and hydrogen bonds in previous courses. This assignment is intended as a review of those concepts. The hydrophobic effect is included, with the understanding that most students have not been exposed to this concept in a prior class. What the students learn about the hydrophobic effect during their pre-activity work will be reflected in their responses during the in-class work. Students also tabulate the strength and distance over which these forces act. The written descriptions and tabulated values are intended to compare and contrast these forces.
The carbon-carbon covalent bond is included in the pre-activity to provide students with a sense of scale or perspective. In general chemistry students are taught that “strong” hydrogen bonds are responsible for the higher than expected boiling point of water. While in organic chemistry, students are told that carbon-carbon bonds are likewise “strong”. The pre-activity forces the students to compare the actual bond energy values associated with both of these strong forces.
For the in-class lesson, students are instructed to form groups of three or four. I am fortunate to have classes of about two dozen students in a room with six tables and movable chairs. Once the groups are established, I pass out copies of the lesson worksheet (S4.) Instructors will find an answer key for the lesson in the supplemental information (S5). Early in the lecture period, I move among the groups checking that students have completed the pre-activity assignment. Over the years, I have decided that it is more important to be open to student questions than immersed in pre-activity grading. So, I generally focus on a limited set of items (e.g. their response regarding hydrophobic effect and completion of the table) and give students full credit for a good faith effort. Scores are recorded in a spreadsheet. Fortunately, my classes are small enough that students that add during the first week of the semester can be dealt with individually. Students start the lesson by reviewing their pre-activity responses in part A. The groups can tend to get bogged down in the discussion of the non-covalent interactions, as their previous exposure to them in prior courses was limited to simpler molecules. I tell the students that the next lecture will review these concepts. This assurance frees students from the worry that they need to master these concepts at this point. In part B, students decide whether or not an interaction is possible for the serotonin molecule. In particular, for the hydrophobic effect, students are specifically instructed to consider the aromatic benzene ring within the serotonin molecule. Students should realize that London dispersion, dipole-dipole, hydrogen bonding, ionic interactions, and the hydrophobic effect are all possible for the serotonin molecule. Again, it is important that students not get too bogged down in details. The covalent bond is presented in the pre-activity for a sense of perspective. I have removed it from the in-class lesson because in my experience students want to find a way to use the strongest force available. In part C, students assess the hydrogen bonding possibilities for the serotonin molecule. To this point, the students have dealt with serotonin itself. The lesson now shifts to consideration of the binding pocket.
In part D, students grapple with the issue of which non-covalent interactions are most appropriate for positions A, B, and C in the binding pocket diagram. In making this decision, the students create a binding pocket that favors the serotonin structure. Students are instructed to choose the strongest force possible at each position.
The student choices for position A are usually either hydrophobic effect or London dispersion force. I find that groups uncomfortable with their understanding of the hydrophobic effect will choose the the London dispersion force because it is more familiar. This question provides a check on their understanding of the hydrophobic effect and an opportunity to discuss it with them. Students can successfully complete the remaining lesson questions with a choice of either hydrophobic effect or London dispersion force at position A.
Groups almost unanimously chose a hydrogen bonding interaction at position B. Some groups go further to specify a hydrogen-bonding acceptor group.
Groups also struggle between two choices for position C. Some groups choose an ionic interaction at this position, while other groups chose a hydrogen bonding interaction. Since I do not teach “ion-dipole” as a separate concept, the students are given the choice between these two similar strength forces. The ambiguity in the choice for position C is not an issue for the continuation of the lesson.
Once they decide on their choices in part D, students are told to keep these choices for the remainder of the lesson. It is important that the choices remained fixed because the next three questions (parts E, F, and G) involve position changes for the serotonin molecule and distance effects for the non-covalent interactions. The goal is no longer to create the best binding pocket for serotonin, but instead discover how changes in the serotonin (ligand) conformation, charge, or orientation affect binding to the pocket that does exist. Most student groups recognize that each of these changes will weaken the binding of serotonin to their designed binding pocket.
The last portion of the lesson (part H) explores the binding of other molecules in place of serotonin. Again, it is important that students continue to use the forces chosen for the initial serotonin-binding pocket. Dopamine is used as an example of another neurotransmitter molecule. Students usually recognize that, although similar functional groups are present, the distances for positions B and C are longer. The longer distances suggest weaker interactions and therefore weaker binding of the alternate neurotransmitter. Glucose is a molecule that favors hydrogen-bonding interactions. Students usually recognize that glucose is less likely to bind due to the disruption of the interactions in positions A and C. The possibility of tryptophan binding in place of serotonin is intended to challenge students understanding. Tryptophan presents the same interactions as serotonin at positions A, B, and C. Therefore, tryptophan is expected to bind to the receptor as well as serotonin does. The last question instructs students to consider how a serotonin binding pocket might have evolved to prevent binding of tryptophan while still allowing serotonin to bind. I use this question in part to keep the fast groups busy while the slower groups are still completing the exercise. There are two common responses to this challenge both involve the tryptophan carboxylate group. Students can choose to put a negatively charged group in the pocket to create an electrostatic repulsion. Other student groups will go with a steric bulk argument suggesting a binding pocket that is too small to accommodate the larger tryptophan molecule.
SUMMATIVE ASSESSMENT
Exam questions using binding pocket diagrams are provided (S1). I use exams that are two-thirds closed book and one-third open book. In the beginning, I used the binding pocket questions in the open book section. Once I had created enough examples for the students, I was able to quickly move this material to the closed book portion of the exam. The early exam questions focused on the non-covalent interaction at a specific position and the relevant amino acid side chains. As students became more proficient with the concepts, I generated questions that were based on site-directed mutations to binding sites from literature reports (see S1 for exam 1 questions from 2014 and 2015).
TEACHING DISCUSSION
Students create or design a binding site for the serotonin molecule through their choices of non-covalent intermolecular forces at positions A, B and C in the binding pocket. The lesson challenges the students understanding of their binding pocket by presenting them with variations of the original serotonin molecule. A rotational conformer of serotonin presents the opportunity to explore how changing molecular orientation may affect the binding of serotonin. This concept is further explored when a flipped orientation of the serotonin molecule is presented. The high pH form of serotonin illustrates how changing molecular charge can affect binding. Both sites B and C are potential hydrogen bonding sites and consideration of hydrogen bond donor and acceptor functional groups comes into play. Student performance on exam questions demonstrates that they are able to successfully design binding sites for other metabolite molecules.
I have presented this lesson orally at the Biochemistry Core Collaborators Workshops organized by Professors Vicki Minderhout and Jennifer Loertscher as part their NSF Teaching in Undergraduate Biochemistry Education grant. The response has been remarkably positive and led to interesting discussions regarding improvements and adaptations.
The choice of interactions presented in the pre-activity is based on a table found in the textbook (8) used by the author. Professor Bruce Heyen (personal communication) has suggested that the “ion-dipole” interaction be discussed. This choice in particular simplifies the response for the binding interaction at position C. Instructors are free change the list of non-covalent interactions to match their course goals and materials. I once used the concept of a pi-cation interaction as the basis for an exam question. Other instructors may wish to teach the pi-cation interaction from the beginning. However, I find that there can be a temptation to try to teach “everything at once.” Of course, this approach will quickly overwhelm the students.
Professors Kathrine Frato and Vicki Minderhout (personal communication) adapted the lesson to include literature data for the binding of LSD to the serotonin receptor. This modification added a quantitative aspect to the lesson. Because Frato and Minderhout had previously introduced amino acid structures, students were prepared to discuss the effect of a mutation to an amino acid used in the binding site.
SUPPORTING MATERIALS
- S1. Serotonin in the pocket: Binding Pocket exam questions
- S2. Serotonin in the pocket: Pre-activity assignment
- S3. Serotonin in the pocket: Preactivity answer key
- S4. Serotonin in the pocket: Guided Inquiry Lesson
- S5. Serotonin in the pocket: Lesson answer key
ACKNOWLEDGMENTS
I would like to thank the students that have used this lesson in my classes. In addition, the NSF grant funded Biochemistry Core Collaborator’s Workshops facilitated by Professors Vicki Minderhout and Jennifer Loertscher provided valuable feedback from fellow biochemistry instructors.
References
- Loertscher J, Green D, Lewis JE, Lin S, Minderhout V. 2014 Identification of Threshold Concepts for Biochemistry. CBE-Life Sciences Education. 13: 516-528.
- Loertscher J. 2011 Threshold Concepts in Biochemistry. Biochemistry and Molecular Biology Education. 39: 56-57
- Wright A, Provost J, Roecklein-Canfield JA, Bell E. 2013 Essential Concepts and Underlying Theories from Physics, Chemistry, and Mathematics for "Biochemistry and Molecular Biology" Majors. Biochemistry and Molecular Biology Education. 41: 302-308
- Tansey JT, Baird Jr. T, Cox MM, Fox KM, Knight J, Sears D, Bell E. 2013 Foundational Concepts and Underlying Theories for Majors in "Biochemistry and Molecular Biology". Biochemistry and Molecular Biology Education. 41: 289-296.
- Brooker RJ, Widmaier EP, Graham LE, Stilling PD. 2017 Biology, 4(th) Edition. McGraw Hill Education. New York, NY.
- Kotz JC, Treichel PM, Townsend JR, Treichel DA. 2015 Chemistry & Chemical Reactivity, 9(th) Edition. Brooks/Cole CENGAGE Learning. Belmont, CA.
- Bissantz C, Kuhn B, Stahl M. 2010 A Medicinal Chemist's Guide to Molecular Interactions. Journal of Medicinal Chemistry. 53: 5061-5084.
- Garrett RH, Grisham CM. 2013 Biochemistry, 5(th) Edition. Brooks/Cole CENGAGE Learning. Belmont, CA.
- Snyder PW, Mecinovic J, Moustakas DT, Thomas III SW, Harder M, Mack ET, Lockett MR, Héroux A, Sherman W, Whitesides GM. 2011 Mechanism of the hydrophobic effect in the biomolecular recognition of arylsulfonamides by carbonic anhydrase. Proceedings of the National Academy of Science 108: 17889-17894.
- Stierand K, Rarey M. 2007 From Modeling to Medicinal Chemistry: Automatic Generation of Two-Dimensional Complex Diagrams. ChemMedChem. 2, 853 - 860.
- Stierand K, Rarey M. 2010 Drawing the PDB: Protein-Ligand Complexes in Two Dimensions. ACS Medicinal Chemistry Letters. 1, 540-545.
- Tian Y, Suk D-H, Cai F, Crich D, Mesecar AD. 2008 Bacillus anthracis o-Succinylbenzoyl-CoA Synthetase: Reaction Kinetics and a Novel Inhibitor Mimicking Its Reaction Intermediate. Biochemistry. 47: 12434-47.
Article Files
Login to access supporting documents
Serotonin in the Pocket: Non-covalent interactions and neurotransmitter binding(PDF | 137 KB)
S1.Serotonin in the pocket exam questions.docx(DOCX | 345 KB)
S2.Serotonin in the pocket-Pre-activity assignment .docx(DOCX | 112 KB)
S3.Serotonin in the pocket-Pre-activity assignment answer key .docx(DOCX | 128 KB)
S4.Serotonin in the pocket-Guided Inquiry Activity .docx(DOCX | 281 KB)
S5.Serotonin in the pocket-Guided Inquiry Activity Answer Key .docx(DOCX | 288 KB)
- License terms
Comments
Comments
There are no comments on this resource.