A Lesson on Matter and Energy at the Organismal Scale: Linking Patterns and Processes Across Diverse Taxa
Editor: Nathan Emery
Published online:
Abstract
Pathways and transformations of energy and matter (PTEM) are a conceptually challenging but essential component of biological literacy. Curricular gaps about PTEM nevertheless remain; although lessons targeting PTEM at small scales (chemical, molecular, cellular) and large scales (ecological, global) are most common, they do not necessarily address understanding of the intervening organismal scale. Moreover, at the organismal scale, student reasoning about PTEM in one taxonomic category (e.g., animal growth) does not necessarily transfer to others (e.g., fungal growth). Building on prior studies, we developed five organismal-scale activities about animals, plants, and fungi that address misconceptions, promote active learning, and require minimal background knowledge of chemistry. These activities are best suited for large (>200) active-learning courses for non-majors or majors with heterogeneous science concept mastery. The lessons involve answering 17 clicker questions, making predictions, building explanatory models, and correcting common misconceptions. The activities include: (i) introducing matter and energy, (ii) understanding what is (and is not) food, (iii) predicting change in mass across the tree of life, (iv) explaining changes in matter and energy using conceptual models, and (v) correcting misconceptions about matter and energy. Pre-post assessments produced significant (p <.01) and meaningful (Cohen's d = -1.8) learning gains, as well as widespread improvement (98% of 449 students). Instructors may choose to use the five activities in isolation or together, or independently make use of the clicker question set. For more advanced students, the activities may be used as pre-course assignments in preparation for more complex learning tasks.
Primary image: Six examples of plants and animals undergoing changes in mass, used for creating conceptual models about matter and energy in organisms. Plant examples have different light environments, and animal examples have different activity levels.
Citation
Kinlock NL, Foley CM, Sbeglia GC, Nehm RH. 2020. A lesson on matter and energy at the organismal scale: Linking patterns and processes across diverse taxa. CourseSource. https://doi.org/10.24918/cs.2020.42
Society Learning Goals
Ecology
- Matter and Energy in Ecosystems
- How does matter and energy move in an ecosystem?
- How do organisms mediate the movement of matter and energy through ecosystems?
- How do organisms obtain and use matter and energy to live and grow?
Lesson Learning Goals
- Students will link observable patterns (e.g., growth, mass increase or decrease) in diverse lineages (i.e., plants, animals, and fungi) to unobservable biological processes (i.e., photosynthesis, cellular respiration, and digestion) at the organismal level (aligns with Ecological Society of America Four-Dimensional Ecology Education Framework D.2).
- Students will connect everyday notions of food with chemical concepts in order to explain how food is used by organisms to carry out biological processes.
- Students will engage in the practice of scientific modeling by building explanatory models that link patterns and processes about matter and energy.
Lesson Learning Objectives
Activity 1: Introducing Matter and Energy
- Students will be able to determine if they hold misconceptions about matter and energy in organisms.
- Students will be able to reason about biological systems by tracing matter and energy in organisms separately.
Activity 2: Understanding What is (and is not) Food
- Students will be able to connect everyday, macroscopic notions of food with chemical concepts (e.g., bonds, molecules).
- Students will be able to compare and contrast food and non-food for plants, animals, and fungi and explain what characteristics make something food for living organisms.
Activity 3: Predicting Mass Change Across the Tree of Life
- Students will be able to use their understanding of photosynthesis and cellular respiration to accurately predict patterns of mass change in plants, animals, and fungi.
- Students will be able to qualitatively predict how biological processes in a plant, animal, or fungus will contribute to the amount of carbon dioxide and oxygen in the air over time in open and closed environments.
Activity 4: Explaining Changes in Matter and Energy Using Conceptual Models
- Students will be able to integrate concepts about matter and energy across different biological scales.
- Students will be able to build conceptual models capable of explaining patterns of matter movement (e.g., mass gain, loss) in different organisms (e.g., plants, animals) and contexts (e.g., sunny and cloudy environments, active or sedentary behaviors).
Activity 5: Correcting Misconceptions about Matter and Energy
- Students will be able to identify and correct common misconceptions about matter and energy in plants, animals, and fungi.
Article Context
Course
Article Type
Course Level
Bloom's Cognitive Level
Vision and Change Core Competencies
Vision and Change Core Concepts
Class Type
Class Size
Audience
Lesson Length
Pedagogical Approaches
Principles of How People Learn
Assessment Type
INTRODUCTION
Pathways and Transformations of Energy and Matter (PTEM) has been identified as a core concept central to biological reasoning, yet it has been shown to be conceptually challenging for undergraduate students for more than 30 years (1,2,3). PTEM is one of the five core concepts essential for biological literacy according to the American Association for the Advancement of Science's (AAAS) Vision and Change report (4). The Next Generation Science Standards (NGSS) for K-12 students also highlight PTEM as Core Ideas for the life sciences (sub-ideas LS1.C: Organization for matter and energy flow in organisms and LS2.B: Cycles of matter and energy transfer in ecosystems; 5). In the Four-Dimensional Ecology Education framework developed by the Ecological Society of America (ESA), PTEM is one of four cross-cutting themes (6). Additionally, PTEM is featured in three learning goals in the Ecology Learning Framework developed as a collaboration between ESA and CourseSource (7). Despite widespread agreement about the importance of PTEM for undergraduate biology education, as of 2020 there are only two relevant lessons on CourseSource (8,9).
Using core concepts to reason across biological scales and throughout the tree of life is a fundamental feature of thinking about living systems (10). Nevertheless, it remains a core challenge for students across many life science domains (e.g., genetics, evolution). For example, learners may develop conceptual understanding at a particular biological scale (e.g., respiration at the molecular scale) but be unable to conceptually connect these processes to related phenomena at other scales (e.g., weight loss at the organismal scale; see work in genetics by 11, evolution by 12, and matter and energy by 1). Alternatively, students may be able to develop conceptual understanding at the organismal scale within a particular taxon/lineage (e.g., animals) but fail to abstract understanding beyond the exemplar instance (e.g., plants; see work in genetics by 13, evolution by 14). Thus, reasoning across biological scales and biological diversity emerge as fundamental learning goals for all of the core concepts of biological literacy (e.g., Pathways and Transformations of Energy and Matter in Vision and Change; 4,10).
Year-long introductory biology courses in the United States are often organized by biological scale and biological diversity: one semester often focuses on molecular and cellular-level phenomena (e.g., cell processes, molecular genetics) with minimal emphasis on diversity, whereas the other semester often focuses on the organismal and ecological scales with targeted attention to patterns and process across the tree of life (e.g., evolution, phylogenetics, ecology). This separation poses inherent challenges for educators seeking to foster coherent biological understanding across scales and lineages for all of the core concepts outlined in Vision and Change. For example, respiration and photosynthesis may be taught in the cellular-focused semester, whereas the carbon cycle may be taught in the organismal and ecological-focused semester, with minimal attention directed to the intervening organismal scale. More materials and learning opportunities are needed for students to reason about PTEM at the organismal scale.
Similar to what other studies have found (e.g., 1), as we began to explore our students' understandings of the core concept of PTEM in the organismal diversity semester of introductory biology, we encountered several challenges: First, we documented a substantial array of widespread misconceptions about PTEM (most of which were documented in prior studies, e.g., 1; see Table 1). Second, in-class discussions revealed that some students possessed fluency with chemical and cellular terms, representations, and discourse practices, whereas others were not familiar with them at all. Third, we found that students that completed introductory chemistry and/or the molecular and cellular introductory biology course prior to taking our course did not display appreciably different performances on matter and energy reasoning tasks at the organismal level compared to students who had not completed these courses (Sbeglia and Nehm, unpublished; see also 1). This latter finding suggested that students' chemical and molecular knowledge did not transfer to the organismal scale, and that additional focus is needed on reasoning across biological scales (see also Nehm 2019). At our university (and others within our public university system), the semester-long introductory biology courses may be taken in different orders; introductory chemistry is only a prerequisite for the semester-long introductory molecular and cellular course but not for the semester-long introductory organismal and evolutionary biology course.
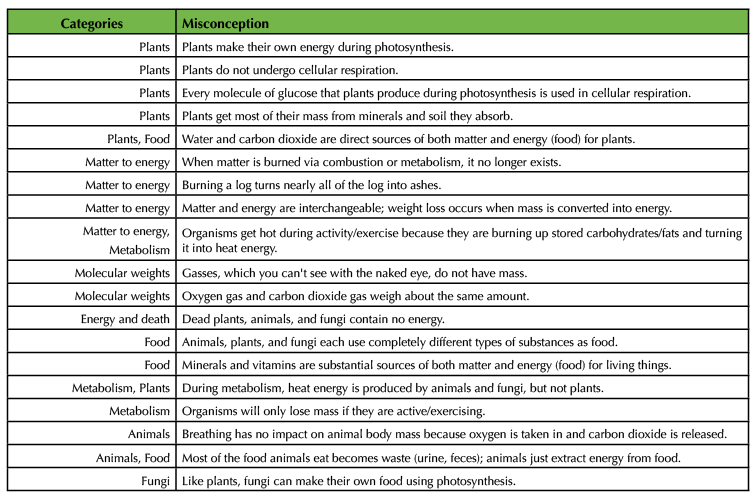
Table 1. List of common PTEM misconceptions gathered from formative assessments conducted over the course of 12 semesters of an introductory biology course with majors and non-majors.
The challenges we faced were perhaps unsurprising given that (i) our course includes a mixture of biology majors and non-majors, (ii) our students may take the year-long introductory biology sequence in different orders, and (iii) prior research on student learning has shown that prior biology and chemistry instruction may not displace misconceptions or alter reasoning about PTEM (e.g., 1). Overall, our semester-long introductory organismal and evolutionary biology course is characterized by: a heterogeneous student population in terms of prior exposure to and understanding of chemical and cellular ideas, widespread misconceptions, and minimal active learning materials focusing on organismal patterns. In order to foster learning within this context, we required course materials that: focused on the organismal level (see comment above); addressed patterns and processes in animals, fungi, and plants; avoided detailed chemical and cellular concepts; addressed misconceptions; were brief (a few classes) and could be implemented in large (250-570 student) classes. Our lesson and associated activities were built with these constraints in mind, but leveraged several pioneering research studies and educational resources on PTEM developed over the past 30 years.
Existing Resources
Some of the conceptual challenges that we faced have been addressed in research-based educational resources, including teaching activities developed to pair with the Diagnostic Question Clusters (DQC,15-17) and the Carbon: Transformations in Matter and Energy (Carbon TIME) materials (18) developed as part of the Environmental Literacy Project at Michigan State University. Our materials overlap with these resources in that they address similar misconceptions about PTEM (e.g., Table 1) and utilize distractor-driven formative assessment questions and activities designed to engage with students' prior knowledge. Our materials differ from these resources in that they: target more basic levels of understanding; focus sustained attention on parallel macroscopic phenomena across plants, animals, and fungi; involve 2-3 class periods; and are amenable to large lecture courses. We discuss more specific differences below.
The DQC activities engage with PTEM at the community, ecosystem, and global scales. With the exception of the DQC's "von Helmont" and "mice in a box" activities, students must have prerequisite understanding of PTEM at the molecular and cellular scales. The activities in the DQC build on this prerequisite knowledge by addressing the interrelationships among different organisms at the ecosystem or global scales. Unlike the DQC activities, the activities involved in the Carbon TIME program do not require extensive prerequisite knowledge. However, the activities are designed in a sequential, scale-based framework that begins with a unit on non-biological chemical reactions, continues with several organismal-scale units, and ends with units at the ecosystem and global scales. In total, the Carbon TIME program requires approximately 40 classroom hours and involves wet labs that take place over several days or weeks (e.g., growing plants, fungi, or observing chemical reactions). This long-term sequential structure is not readily adaptable to undergraduate courses in which the entire semester includes 37 hours of class time.
Following instruction in the foundational unit, all Carbon TIME units require knowledge of types of chemical bonds and balancing chemical equations, and they include activities in which students carry out chemical reactions by manipulating physical molecular models. The DQC does not require detailed knowledge of chemistry in activities and does not include lecture materials. Our activities approach molecular structures and molecular processes as a way to understand organism-level patterns, but students are not expected to know chemical structures or how to formally balance chemical equations.
As previously mentioned, there are two lessons available on CourseSource that target PTEM learning goals, but these lessons differ from ours in scope and focus. Smith and colleague's (8) lesson focuses on PTEM in photosynthetic organisms, and Darby and Goodwin's (9) lesson involves PTEM at the ecosystem scale. Instructors interested in additional reading about PTEM are encouraged to consult these sources: Anderson et al. (1), Wilson et al. (2), and Mohan et al. (3), and Maskiewicz et al. 2012 (15).
Lesson Activities
Our lesson includes five active-learning activities (Table 2). These activities focus on PTEM in animals, plants, and fungi and collectively are designed to sustain attention on organismal patterns prior to moving on to the ecology unit. Unsurprisingly, we have found that until students demonstrate mastery of organismal-level patterns and processes relating to PTEM, they are not able to successfully reason about ecosystems and global cycles (e.g., carbon). Our materials include short videos (~3-5 minutes), numerous collaborative clicker questions, prediction and modeling activities, and misconception identification and correction tasks (Table 3). All materials are designed to engage with students' prior knowledge and misconceptions, and all activities are designed to be implemented in permanent, small, collaborative student groups (3-4) within a large lecture course.
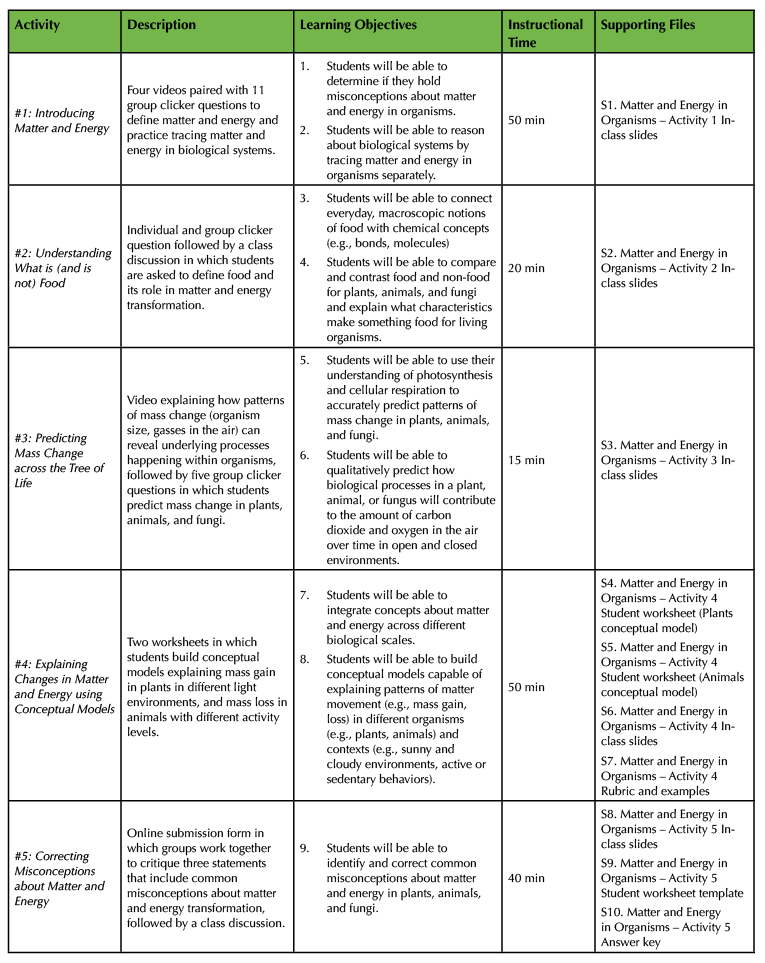
Table 2. Summary of five PTEM activities.
In Activity 1, students are introduced to PTEM in a series of four brief (~3-5 minutes) videos that are each paired with collaborative questions (Supporting File S1. Matter and Energy in Organisms – Activity 1 In-class slides). The videos provide foundational, misconception-focused (Table 1) content about PTEM, and are suitable for students with diverse science backgrounds (e.g., non-majors, majors). This activity can be situated within the conceptual framework developed as a part of the Biology DQC, as students are introduced to the overarching principles of conservation of matter and energy using processes involved in the generation, transformation, and oxidation of organic carbon compounds. In Activity 2, we ask students to reason about food, the building block of mass gain, from the perspective of PTEM. Students then engage in group clicker questions and structured class-wide discussions (Supporting File S2. Matter and Energy in Organisms – Activity 2 In-class slides). In Activity 3, we ask students to apply the content knowledge presented in the first activity in order to predict changes in mass in diverse organisms (plants, animals, and fungi) over a short time period via the generation (photosynthesis), transformation (metabolism), and oxidation (cellular respiration) of organic carbon (Supporting File S3. Matter and Energy in Organisms – Activity 3 In-class slides). In Activity 4, students integrate their knowledge from the previous activities by building conceptual models explaining patterns of mass change over time in different organisms (plants and animals) and contexts (sunny and cloudy environments, active and sedentary activity levels) (Supporting File S4-5. Matter and Energy in Organisms – Activity 4 Student worksheets, Supporting File S6. Matter and Energy in Organisms – Activity 4 In-class slides). Students are given a set of concepts to incorporate into their models, including key molecular processes (i.e., cellular respiration, photosynthesis, digestion). In Activity 5, students critique and revise statements about PTEM that include multiple common misconceptions (Supporting File S8. Matter and Energy in Organisms – Activity 5 In-class slides, Supporting File S9. Matter and Energy in Organisms – Activity 5 Student worksheet template).
Intended Audience
These activities were carried out in large-enrollment (>200 students) undergraduate introductory biology courses for biology majors and non-majors. Non-majors are a heterogeneous population of STEM and non-STEM students; there is no separate general education biology course at the university and not all students enroll in this specific science course. Versions of these activities have been carried out in previous years beginning in 2017. Students in these courses were at different points in their sequence of courses for their respective majors. Students came from very different backgrounds within the United States and internationally, and only some of the students have taken high-school or undergraduate chemistry courses.
Required Learning Time
These five activities, if used in sequence (along with supplementary lecture materials), are designed to take approximately 160 minutes. In our course, they took slightly longer than two 80-minute classes (one week). However, these activities are designed so that instructors can use them separately, as pre-course materials in flipped classroom settings, or as basic review tools for more advanced courses.
Prerequisite Student Knowledge
Our activities were designed for students with very limited scientific proficiency in order to provide opportunities for all students to get up to speed and successfully engage in reasoning about PTEM. Students with little background in chemistry or cellular or molecular biology are able to successfully meet the learning objectives (see below). Students should have a middle school understanding of what molecules, chemical reactions, and chemical bonds are, and should be familiar with symbolic representations of carbon, oxygen, and nitrogen (e.g., aligning with New York State P-12 Science Learning Standards PS1.A-B). Understanding very basic information about plants, animals, and fungi is helpful. In our course, the unit about the diversity of life, in which the phylogeny and characteristics of plants, animals, and fungi are taught, occurs prior to the PTEM unit. Prerequisite knowledge about the nature of science (and science practices such as modeling and explanation) is also important. Specifically, for Activities 1 and 4, students should be familiar with the idea of observable patterns and unobservable processes, and for Activity 4, students should be familiar with the epistemic aims of scientific modeling (i.e., to further understanding, explain phenomena). We provide a few slides that instructors could use to introduce these topics, if needed. For Activities 3, 4, and 5, students should have middle school level understanding of photosynthesis and cellular respiration (e.g., aligning with NYS P-12 Science Learning Standards LS1.C). Specifically, for photosynthesis, students need to know that the inputs are carbon dioxide and water molecules; the outputs are glucose (a sugar), oxygen gas, and water molecules; and that sunlight provides the energy for the chemical reaction to occur. For cellular respiration, students need to know that large, high-energy molecules (sugars) are used as a source of matter and energy (i.e., as food), and that the chemical reaction requires oxygen and carbon dioxide and water are outputs. Finally, students need to know how organisms build and add tissue to their bodies, and that some of the molecules extracted from food are used in storage and structural molecules (e.g., there is not a one-to-one relationship between photosynthesis and respiration). In our course, we provide brief lecture slides to supplement our active learning materials.
Prerequisite Teacher Knowledge
The instructor should have understanding of PTEM at the level of a non-majors introductory biology textbook. Specifically, the instructor should understand the general features of cellular respiration, photosynthesis, digestion, and general organismal metabolism (e.g., sugars to storage/structural carbohydrates or fats, and vice versa). The instructor should understand the phylogenetic relationships and basic ecology of animals, plants, and fungi. Instructors should be familiar with the nature of science concepts of observation, inference, pattern, process, model, modeling, and explanation.
SCIENTIFIC TEACHING THEMES
Active Learning
Students are expected to work in groups of three to four for all activities. In our courses, we had undergraduate teaching assistants (UGTAs) who were prepared to help foster effective communication, problem-solving, and teamwork in small groups. In the first three activities, students collaboratively discuss and answer clicker questions based on video and lecture material. In the fourth activity, students create two conceptual models. In the fifth activity, students write three short critiques of statements involving misconceptions about PTEM. In full class discussions during or following these activities, we "cold call" groups (via random selection) to ensure equal representation and encourage engagement.
Assessment
To assess student learning, we designed and administered an instrument called the Movement of Matter in Organisms (MOMO, see 19). The instrument contains 60 multiple-choice and multiple-answer items (20 plants, 20 animals, and 20 fungi), several of which were derived or modified from prior assessment items (e.g., 1; DQC, 15-17). The instrument was presented in a multi-matrix format using a complete matrix booklet design (five blocks and five test forms, balanced for block order) (see 20). Test forms contained 36 items and were designed to be balanced across organismal contexts (animals, plants, and fungi) and learning objectives (see Table 2). Each student received the same test form pre- and post-instruction. The items focus on the movement of matter under a variety of conditions (open and closed systems, living and dead organisms, active and inactive organisms). The items are focused on the organismal scale, but the answer options include processes at the molecular scale. Items include common misconceptions about matter and energy as distractors. Several items ask respondents to make and explain predictions about changes in the mass of biological systems over time. Other items ask students to critique naive statements about PTEM. The instrument was administered online about a week before and after the lesson occurred.
Inclusive Teaching
The course in which this lesson took place was centered on inclusive and equitable teaching practices. Many approaches were implemented to make the course more personal, structured, and engaging, in line with recommendations for building an inclusive environment suitable for all learners (21).
The design and delivery of curricular materials was based on evidence about student reasoning, and the impact of diverse backgrounds and prior experiences on learning. All classes incorporated collaborative group work conducted in small, permanent groups (3-4 students). The groups were engineered to be diverse in terms of students' prior coursework, majors, and demographic variables. Diverse groups were generated using the "create_groups" function in the R package 'minDiff' v. 0.01-3 (22). This function assigns students to N groups by minimizing (via 10,000 repetitions) the differences among groups for one or multiple criteria (e.g., prior biology courses, plan, demographic variables). The result is a set of group assignments in which students are heterogenous within groups and homogeneous among groups.
Each group was assigned to work with a UGTA who was responsible for formally documenting group progress and trying to understand thinking in order to foster learning. Each UGTA oversaw a maximum of 35 students. UGTAs were responsible for knowing students' names and seating locations and were able to identify when groups were in need of assistance. UGTAs were utilized to help the instructor gauge student engagement and identify topics that were difficult for students. UGTAs wore name tags and students were provided with name tents. The instructor made efforts to call students by their names in every class, both during small group conversations and during class-wide discussions. The instructor also used transparent and low-stakes "cold calling" to encourage engagement during activities and to foster the contribution of diverse student voices.
To appropriately prepare UGTAs for their important roles, they participated in 4.5 hours of workshops throughout the semester (focusing on cognition, engagement, and diversity) that sought to encourage inclusive teaching practices and a growth mindset. UGTAs were also prepared weekly with information about the upcoming activities, content, and learning difficulties they could expect to encounter.
LESSON PLAN
The five activities in this lesson took slightly longer than two 80-minute lecture periods (see Table 3). However, the lessons are not strictly dependent on one another and instructors may use these activities modularly, as review materials, or to supplement other PTEM course materials.
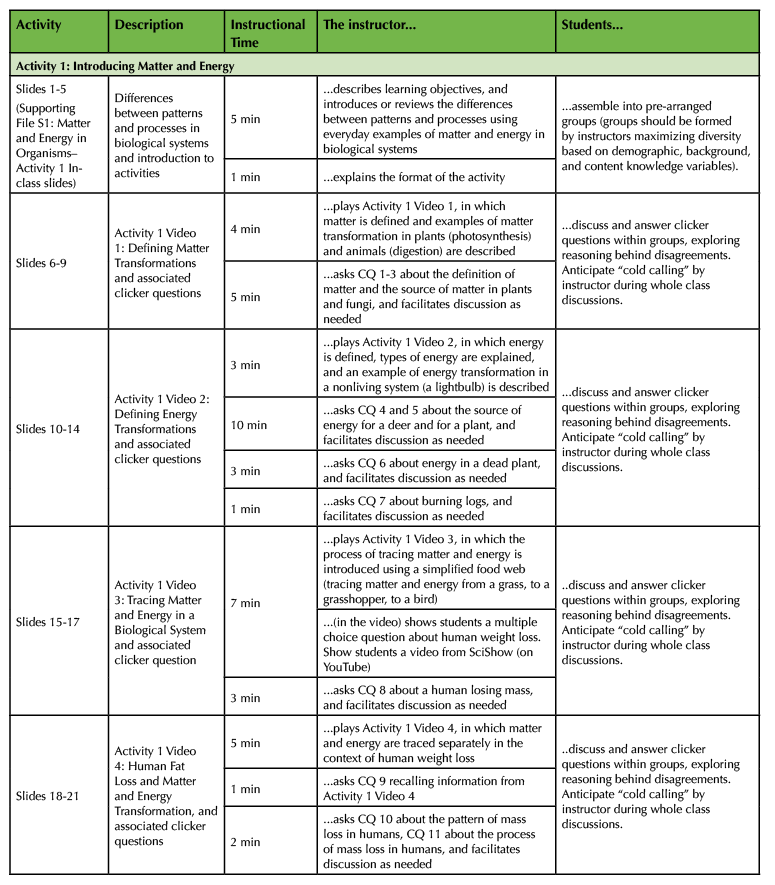
Table 3. Teaching timeline (activity 1).
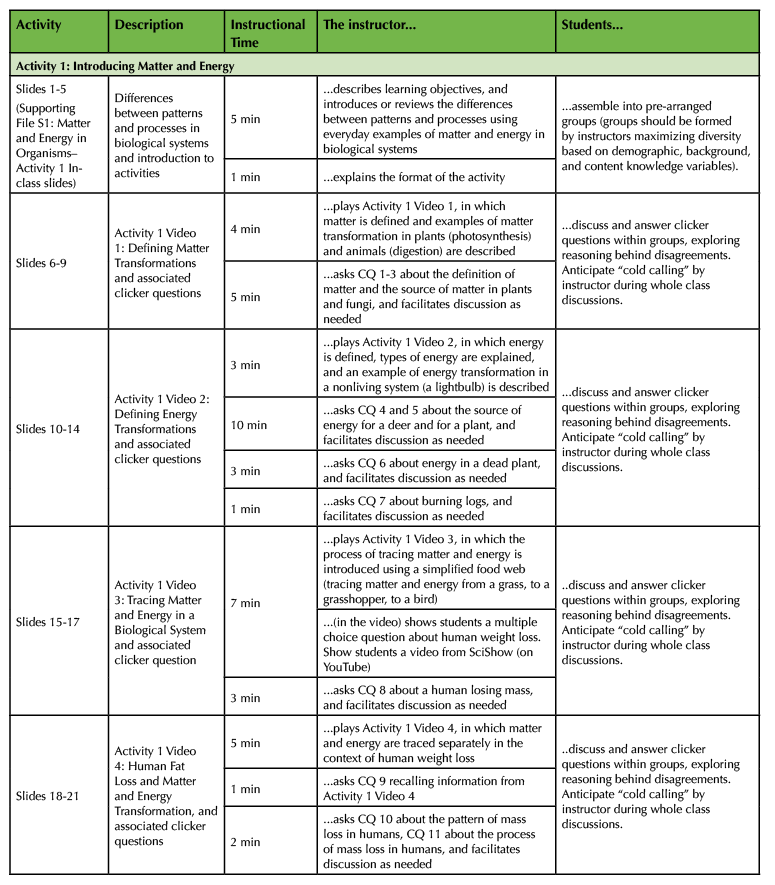
Table 3. Teaching timeline (activity 2 and 3).
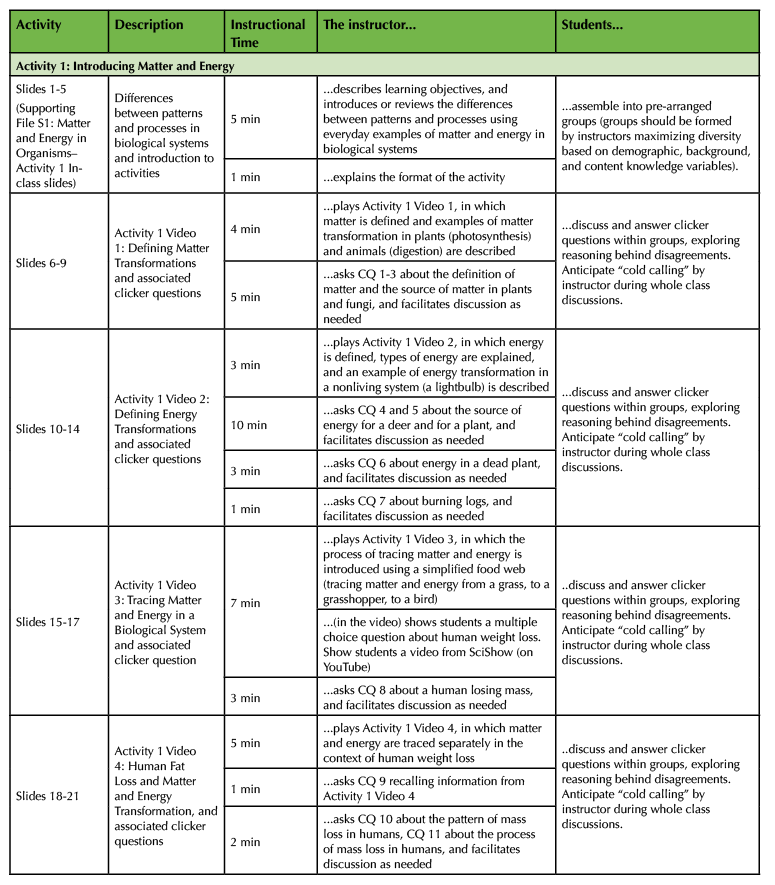
Table 3. Teaching timeline (activity 4 and 5)
Instructor Pre-Class Preparation
Before any activities, the instructor should engineer small groups, maximizing within-group diversity using demographic, background, and content knowledge variables gathered in pre-course assessments (e.g., DQC, 15-17). Instructors should prepare a strategy for group "cold calling" (e.g., we assign numbers to groups and select groups using popsicle sticks). For Activity 4, instructors should print and copy two conceptual model handouts per group. The instructor should review common misconceptions (Table 1) as they are likely to be raised by students in class discussions.
Activity 1: Introducing Matter and Energy
Activity 1 (see Tables 2, 3) introduces very basic ideas about PTEM in biological systems within a pattern-process framework that persists throughout the remaining activities (Supporting File S1. Matter and Energy in Organisms – Activity 1 In-class slides). Linking observable patterns (or phenomena) at the organismal scale (e.g., losing mass, burning logs) with unobservable processes is an important learning goal of this lesson. The first few slides frame biological reasoning and problem solving using the pattern-process distinction. Students are then asked to use this framework to collaboratively solve biological problems about PTEM. Students watch four short videos (~3-5 minutes each) interspersed with clicker questions. The videos review the differences between matter and energy and emphasize the value of tracing them separately in biological systems. We have found that the misconception that matter and energy may be converted into one other is so pervasive and persistent that students will require multiple opportunities to check their reasoning in different problems. Activity 1 Video 1 defines matter and introduces the movement of matter in biological systems, and Activity 1 Video 2 defines energy and introduces energy transformation. Each video is followed by several clicker questions culminating in a structured class-wide discussion. The clicker questions contain common misconceptions as distractors, but are closely aligned with the content in the videos. Activity 1 Video 3 introduces the tracing of matter and energy in a living system (a simple three-species food chain), but emphasizes their separate and non-interchangeable pathways.
Keeping the framework of tracing matter and energy separately in mind, students are asked to consider (but not yet answer) a question about weight loss in humans. As many other studies have found, most students do not understand the processes associated with patterns of weight loss. The question is engaging to most students and produces lively discussions. A YouTube video produced by SciShow (that may be downloaded or streamed, https://www.youtube.com/watch?v=C8ialLlcdcw) introduces the science behind weight loss. A multiple choice version of the weight loss question is then presented for groups to discuss collaboratively. The theme of mass change in humans is continued in Activity 1 Video 4, which models how to trace matter and energy separately in the context of human weight loss. This video is followed by three collaborative clicker questions and a short class-wide discussion.
Activity 2: Understanding What is (and is not) Food
Food is the source of matter and energy for organisms and is therefore essential to understanding how matter and energy move through biological systems. Nevertheless, few students have a biological understanding of food. In this activity, students learn a definition of food that incorporates concepts of matter and can be generalized to plants, animals, and fungi (see Tables 2, 3). The activity begins with a clicker question that asks students to select visual representations of food from a group of images (Supporting File S2. Matter and Energy in Organisms – Activity 2 In-class slides). We present diverse examples, including living and dead plant and animal material, plant nutrient formula (with a "plant food" label), feces, carbon dioxide, water, sugar, and vitamins (e.g., calcium tablets). This clicker question is presented prior to a scientific definition of food and should be asked as a "no penalty" clicker question. Students first decide their answers individually, and then discuss them in groups. After students and groups commit to their answers, a working definition of food and its role in PTEM is introduced in a short set of slides. Specifically, food is defined by its contribution of matter and energy to organisms, and by its characteristic structure of carbon-carbon bonds (non-food includes, for example, minerals, water, and other molecules lacking carbon-carbon bonds). Visualizations of carbon-carbon bonds and examples of carbon-rich and carbon-poor molecules are provided. Photosynthesis is then described as a process that builds the types of molecules that we have defined as food. Groups are shown the molecules (including their molecular structures) involved in photosynthesis and discuss which among the products and reactants could be considered to be "food." This activity aims to connect students' everyday notions of food with chemical and molecular concepts.
Activity 3: Predicting Mass Change Across the Tree of Life
Activity 3 (see Tables 2, 3) involves inferring unobservable processes from observable patterns (i.e., mass change) (Supporting File S3. Matter and Energy in Organisms – Activity 3 In-class slides). Specifically, this activity asks students to apply their knowledge of PTEM in animals, plants, and fungi. The activity begins with Activity 3 Video 1, in which students are shown that the processes of photosynthesis, cellular respiration, and growth can be inferred from (i) measures of carbon dioxide and oxygen in the air surrounding an organism and (ii) changes in the mass of the organism. Students are presented with five scenarios in open and closed systems (e.g., human standing in a box on a scale, plant in high sunlight on a scale, animal sleeping on a scale) and asked to use their knowledge about these processes to make predictions about the patterns they expect to observe over time (e.g., change in organismal mass, CO2 over time). In the closed system example, an organism is placed in a box on a scale such that the gasses being exchanged in the air surrounding the organism (e.g., CO2, O2) are trapped in the box and are thus part of the system being weighed. This scenario addresses the common misconception that air lacks mass. In an open system example, students must consider the consequences of the processes of photosynthesis and respiration for organismal mass. These scenarios are meant to be discussed in groups and predictions are recorded in the five clicker questions. This activity is designed to help students more directly link biological patterns to the processes of respiration and photosynthesis (vs. common existing naive ideas; see Table 1).
Activity 4: Explaining Changes in Matter and Energy Using Conceptual Models
Activity 4 (see Tables 2, 3) asks students to integrate their knowledge about PTEM by collaboratively building conceptual models that explain patterns of mass change over short time scales (i.e., 3 hours). Students build two conceptual models as part of this activity: one about mass change in plants under a variety of light conditions (i.e., low, high) (Supporting File S4. Matter and Energy in Organisms – Activity 4 Student worksheet Plants conceptual model), and one about mass change in animals at a variety of activity levels (i.e., sleeping, standing, running) (Supporting File S5. Matter and Energy in Organisms – Activity 4 Student worksheet Animals conceptual model). The prompt for each model is to explain patterns. First, groups must predict the pattern of change they expect to observe across all cases (i.e., increasing, decreasing, or no change). Second, groups work together to build a conceptual model that explains the pattern. A set of 15 concepts is provided for students to incorporate into their explanatory model. Students are instructed to draw their models similarly to a concept map (e.g., connect the concepts with arrows that reflect the directionality of the relationship, write explanations for every arrow). Clear guiding instructions are essential to the success of this activity and are provided in the PowerPoint slides (Supporting File S6. Matter and Energy in Organisms – Activity 4 In-class slides). Groups are required to check in with the instructor or UGTA once they have predicted the pattern of change, but prior to constructing a model. We recommend that the instructor and UGTAs circulate during this activity and engage with every group. During these one-on-one discussions, students can be prompted to explain their conceptual models, which often reveals misconceptions and missing links. To ensure that all groups have thought deeply about their explanations, we recommend requiring all groups to explain their models to UGTAs or the instructor before submitting it at the end of the activity (one plant and one animal model should be submitted for each group). Our original intent was to have students synthesize their two models into one general model that incorporates all six cases. However, each model took students more time than we expected. We have provided a rubric and several student examples for each conceptual model (Supporting File S7. Matter and Energy in Organisms – Activity 4 Rubric and examples).
Activity 5: Correcting Misconceptions about Matter and Energy
Activity 5 (see Tables 2, 3) is a capstone task that provides students with a final opportunity to reflect upon their misconceptions about PTEM, and work in groups to resolve any remaining confusion. Specifically, groups work together to critique 18 common misconceptions (randomly split into six sets of three) about matter and energy in plants, animals, and fungi. The instructor assigns each group one set of three statements. The instructor will describe the learning objectives for the activity and explain the instructions (Supporting File S8. Matter and Energy in Organisms – Activity 5 In-class slides, Supporting File S9. Matter and Energy in Organisms – Activity 5 Student worksheet template). In our classes, groups submit their work using Google Forms, but many methods could be used depending on instructor preference. In groups, students work together to write short paragraphs that explain whether a statement is correct, partially correct, or incorrect, followed by an explanation of why. We require explanations to be a minimum of 400 characters to encourage substantive reasoning. We recommend that instructors and teaching assistants visit each group at least once during the activity, asking them to explain one or more of their responses. At the end of the activity, the class will come together for a 15 minute discussion of the statements with the instructor randomly calling on groups to explain their narratives. The instructional slides explicitly prepare students for "cold calling" prior to the start of the activity. We provide instructors with an answer key, describing ideal critiques for each statement (Supporting File S10. Matter and Energy in Organisms – Activity 5 Answer key).
TEACHING DISCUSSION
Assessment of Lesson
To evaluate the effectiveness of the lesson, we administered the MOMO instrument in Fall 2019 to a diverse sample (~23% Underrepresented Minority, ~41% non-biology majors) of undergraduate students enrolled in an introductory biology course at a large, public, research-oriented university in the northeastern United States. In total, 449 students completed the MOMO before and after the matter and energy lesson was implemented (92% participation rate). We used Rasch analysis to establish good measurement of the intended construct (23) and to generate latent measures on a linear scale that could be analyzed using parametric statistics (24). Separate Rasch models were run on the pre- and post-instruction survey. To allow the two time points to be meaningfully compared, the post-survey item difficulties were anchored to the pre-survey at model estimation. Eight items (out of 60) did not fit the post-survey or the anchored pre-survey Rasch models and were removed from both time points. Nearly all of the remaining items had acceptable fit (weighted and unweighted mean squares fit [MNSQ] statistics between 0.5 and 1.5 logits) to the Rasch model at both time points (24). The few items that were outside of this acceptable range had a weighted MNSQ of < 2 indicating that although they did not contribute to measurement, they also did not degrade it and were thus retained (24). The item and person reliabilities for the pre- and post-survey models were acceptable (Pre: WLE = 0.69, EAP = 0.71; Post: WLE = 0.79, EAP = 0.84) (25). The eigenvalue of the first contrast in a PCA of Rasch residuals for each form of the test was between 2.89 and 3.45, indicating a slight, but small, departure from unidimensionality (24). Person ability measures were generated using a Warm's Mean Weighted Likelihood Estimation (WLE) of the item difficulty parameters (26).There were significant and large gains in the latent ability of respondents from the pre- to the post-survey (paired t-test: t = -41.85, df = 448, p < 0.001; Cohen's d = -1.8357) (Figure 1). Out of 449 students, only 10 did not improve their latent measure from the pre- to the post-survey. Overall, students' mean probability of answering items correctly nearly doubled from the pre- to the post-survey (i.e., Animal items: 34% in pre to 71% in post; Fungus items: 36% in pre to 69% in post; Plant items: 36% in pre to 73% in post). The increase in mean probabilities from pre- to post-survey was similar among taxa, but was largest for plant items. Overall, the lesson was associated with significant and meaningful learning gains for the challenging topic of PTEM.
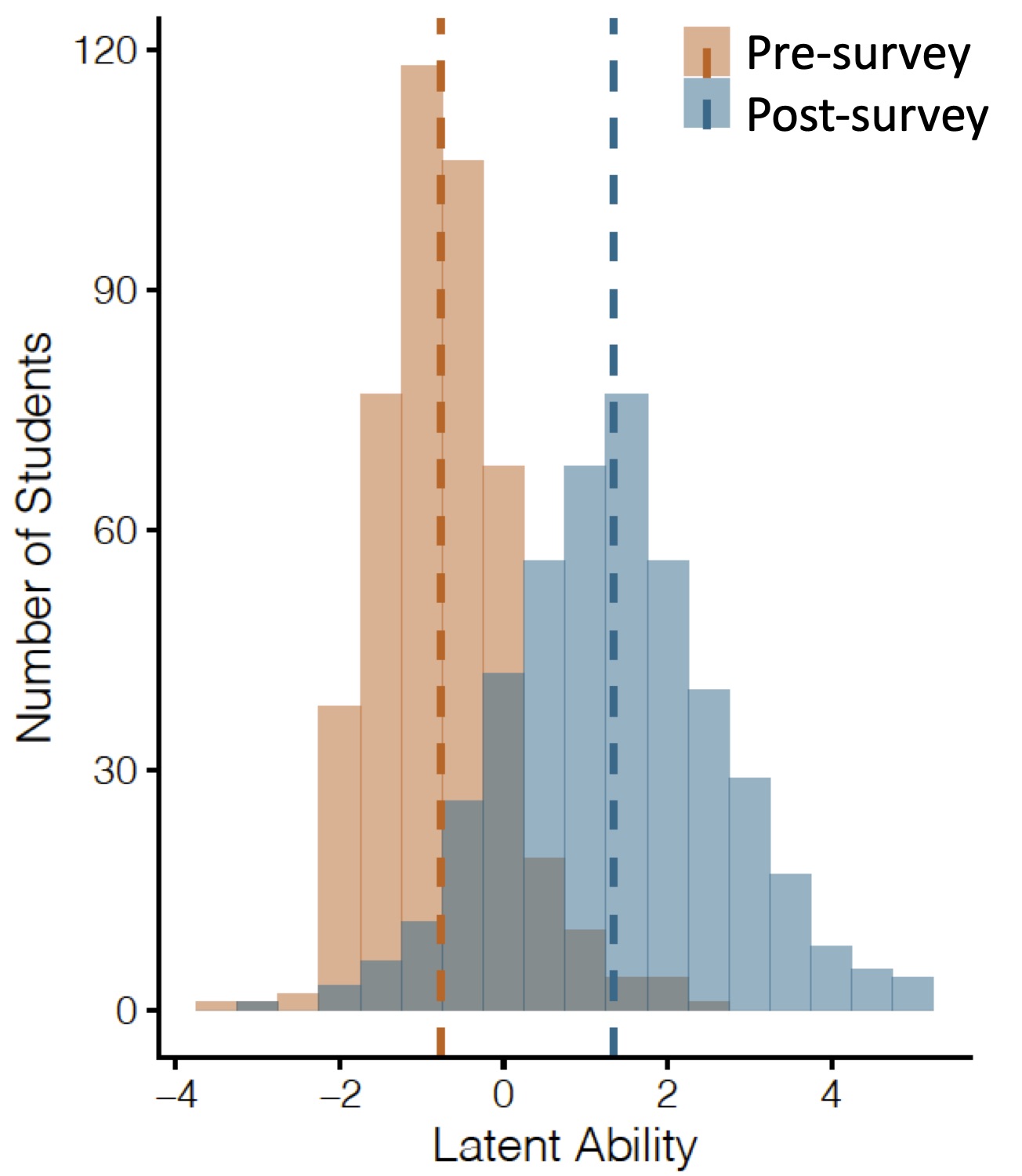
Figure 1. Distribution of student ability measures pre- and post-lesson. The student sample (N = 449) included both majors and non-majors, and represented diverse preparation and demographic backgrounds. The students were enrolled in an introductory biology course at a large, public, research-oriented university in the northeastern United States. The time points are anchored to each other and directly comparable. Dashed lines indicate mean student ability, which may be considered analogous to amount of understanding.
We anticipate that instructors will use the assessment data generated from (i) clicker questions, (ii) model building activities, and (iii) misconception critiques to assess learning. We provide a rubric for the model building activity (Supporting File S7. Matter and Energy in Organisms – Activity 4 Rubric and examples) and an answer key for the misconception critiques (Supporting File S10. Matter and Energy in Organisms – Activity 5 Answer key) to aid instructors in assessment. Readers interested in a more robust assessment are encouraged to contact the authors for access to the MOMO assessment tools.
Instructor Observations and Possible Improvements
Activity 1 (see Tables 2, 3).
As an introductory activity, Activity 1 is designed to help bring all students up to speed on the foundations of PTEM. Most of the collaborative clicker questions in Activity 1 (Supporting File S1. Matter and Energy in Organisms – Activity 1 In-class slides) are very closely aligned with the videos (https://www.stonybrook.edu/commcms/ecoevo/_publications/_downloads/sbeglia), and groups tend to perform well on them (>90% correct for most questions in our class). However, we know from prior experience that many students do not perform well when answering these same questions individually. Therefore, encouraging active discussion and allowing sufficient time for groups to communicate is essential for successfully preparing all students for the subsequent activities in this unit. We encourage instructors to resist the urge to rush this activity, which on its surface may seem much too basic for college-level students. Students hold many misconceptions about PTEM, even when they have excelled in relevant advanced coursework.
One particularly pervasive and persistent misconception is that matter and energy can be converted into each other in biological systems. In every semester in which we have taught this material, at least one student has publicly questioned our assertion that this naive idea is incorrect, and cited Einstein's mass-energy equivalence equation (E=mc2) as evidence. Informal interviews with students have also revealed a sense of annoyance and discomfort with instructional efforts to correct their naive reasoning about the relationship between matter and energy in biological systems. While instructors need not provide a detailed explanation as to why E=mc2 is not applicable to biological systems, it is important that instructors anticipate this argument being raised in their class and should expect that many students hold it even if few verbalize it. In our experience, the following statement is sufficient to address this naive reasoning when it inevitably arises: "While there are conditions in the universe in which matter and energy can be converted into one another, these conditions do not apply to living systems, which are the focus of this class." In recent iterations of this course, we have begun to address this misconception earlier in the semester (during the unit on plants) and have found that this format has improved the initial reception of PTEM material.
There are a few notable patterns in the clicker responses in this unit that instructors should know in advance. While groups tend to perform well on most of the clicker questions, there are two that consistently show reduced performance: CQ 3 and CQ 5. The videos in this activity directly address matter in plants and animals, but do not directly address it in fungi. Students are asked to respond to a clicker question about fungi (CQ 3) and must transfer knowledge about other heterotrophs that are explicitly addressed in the video (i.e., animals) to the fungal case. While most students answered the fungus question correctly, instructors can expect reduced performance on this clicker question as compared to a similar question (CQ 2) about plants. Furthermore, students seemed to struggle with the idea that the sun is, for the most part, the only energy source for plants. After relevant instruction, responses to CQ 5 revealed that about 25% of students classified the sun as the most important, but not the only source of energy for plants; gasses from the air and water and minerals from the soil were considered to have small to medium energy contributions. It is important that this misconception is addressed during Activity 1 because it has implications for students' understanding of the notion of food (the building blocks of matter and energy) for organisms, which is addressed in Activity 2.
Activity 2 (see Tables 2, 3).
This activity aims to connect students' everyday notions of food with chemical and molecular concepts. Students began this lesson with a lot of misconceptions about food, or more specifically, about what is not food. Specifically, in the initial no-penalty individual clicker question (CQ 12), nearly all students in our classes selected the non-food images (e.g., water, CO2, calcium) as frequently as the food images (Supporting File S2. Matter and Energy in Organisms – Activity 2 In-class slides). Understanding what is and is not food is foundational to understanding how organisms, including plants, use food (in the processes of digestion, assimilation, cellular respiration, etc.) to attain matter and energy for growth.
Activity 3 (see Tables 2, 3).
This activity presents several scenarios and asks students to use their knowledge of the processes of photosynthesis and cellular respiration to make predictions about simple systems. This lesson is foundational for building explanatory models of mass change in plants and animals (Activity 4). Although our students performed well on the clicker questions in Activity 3 (Supporting File S3. Matter and Energy in Organisms – Activity 3 In-class slides), they found it challenging to build explanations for these patterns in Activity 4. Therefore, when discussing these clicker questions, we recommend focusing students' attention on explaining the processes underlying each pattern.
Activity 4 (see Tables 2, 3).
This activity requires that students integrate the ideas from Activities 1-3 into conceptual models that predict and explain patterns of mass change over short time scales. This is an inherently difficult task, and the instructor should take steps before and during the activity to increase student success.
First, the instructor should establish realistic expectations for the students. We recommend explicitly discussing the learning objectives of this activity with students and explaining that knowledge integration can be difficult and time consuming. We are explicit with students that struggling with this activity is not a sign of failure or cause of discouragement, but rather an essential step towards learning how all the pieces fit together. Nearly every group will struggle with this activity, and letting students know this in advance may allow them to engage in it using a growth mindset.
Second, our students have difficulty remembering what models are and how they are used in science. We have found that students are able to accurately connect many ideas, but often these ideas do not work together to explain the target phenomenon (e.g., mass increase in plants). For this reason, instructors should review the concepts of pattern and process and how these connect to the epistemic aims of scientific modeling (i.e., furthering understanding of a system, explaining patterns; for a review, see 27). The introductory slides of this activity are designed to help instructors emphasize these points (Supporting File S6. Matter and Energy in Organisms – Activity 4 In-class slides).
Third, we have found that the initial steps of model building are consistently very challenging for students. Our materials include slides that make specific recommendations about how to initiate model building. One fundamental step is to establish and write down the phenomenon (i.e., the pattern) for which an explanation is sought in the center of the worksheet (Supporting Files S4-5. Matter and Energy in Organisms – Activity 4 Student worksheets). This could also be done collaboratively as a class.
Fourth, we recommend that instructors and teaching assistants interact with all groups early on in the activity in order to ensure that they understand the epistemic aims of modeling, and to help groups initiate model construction. In our class, for example, UGTAs made various suggestions to groups about model structure: placing the three organisms at the top of the models, describing cellular respiration and photosynthesis on either side of the plant model, or keeping matter and energy on separate sides of each model. For UGTAs to be successful at engaging with students, we recommend that they build their own models in advance of the activity. We have noticed major differences in our UGTAs' confidence in engaging with students and their proficiency in model building in semesters in which they have and have not spent time building their own models.
Fifth, the instructor should allow enough time for students to fully engage with this activity. If the activity ends too early, students may leave class without making important cognitive breakthroughs, and could feel defeated in the process. When we have determined that students require more time with this activity than we expected, we have returned the models in the next class and devoted additional class time to them. The recommended time for this activity (~45 minutes) is reflective of our observations over multiple semesters.
Sixth, in our experience, it is difficult for students to identify errors in their own models. Some groups recognize that problems exist and seek help to identify them. This is productive behavior that should be encouraged. However, other groups may assume that they have built accurate conceptual models and may reach this conclusion early in the activity. We have identified several such groups in each iteration of this activity. We recommend requiring all groups to explain their models (in their finished or unfinished form) to instructional staff relatively early in the activity so that modifications can be made if necessary. In our experience, this strategy helps students recognize weaknesses and address them in time. We also recommend having additional copies of the worksheet on hand for those groups who decide to start their models from scratch.
This modeling activity has exposed misconceptions that our students hold. Observations from this activity in prior semesters have been incorporated into the activities in this lesson. However, our most recent implementation of this activity exposed additional misconceptions about the relationship between photosynthesis and respiration that our material may not adequately address. Multiple groups did not sufficiently integrate cellular respiration in their models of plant growth, suggesting that the misconception that plants do not respire was retained by numerous students. This may be an especially difficult misconception to completely address. Furthermore, many groups that were able to connect photosynthesis to cellular respiration appeared to depict a one-to-one relationship between these two processes (i.e., every molecule of glucose formed by a growing plant in photosynthesis would be used in cellular respiration by the plant). Instructors may wish to explicitly address this misconception before the modeling activity and include "build and add tissues" to the concept list in the modeling worksheets.
Finally, it is important to note that our original intention was to have students successfully complete the two models and then construct a more complex model that integrated the mass gain and loss in plants and animals. However, in every iteration of the activity, we discovered that the individual models were much more challenging than we expected, and there was not sufficient class time to complete this task. Instead, we have raised the question of how the individual models would differ from a general model and spent time critiquing individual group models.
Activity 5 (see Tables 2, 3).
This activity asks students to use the mental models they have been revising and building in Activities 1-4 to critique and correct naive statements about PTEM (many of which they had at the beginning of class) (Supporting File S9. Matter and Energy in Organisms – Activity 5 Student worksheet template). We observed that groups could quickly identify false statements and provide short explanations. However, it was difficult for groups to elaborate on these explanations and reach the 400-character limit we set on the Google Forms submission form. The character limit encourages substantive explanations and is an important aspect of the assignment. However, instructors should be aware that students may initially be uncomfortable being asked to write such "lengthy" explanations. Multiple students reflected that despite their reservations, the character limit made them think much more deeply about the concepts. The amount of time that we recommended for this assignment was sufficient for most of our groups, but some did not reach the character limit in the allotted time. To allow all groups to submit their work and receive credit, we removed the character limit from the online submission form at the very end of the activity so that answers with fewer than 400 characters could be submitted.
There are many possible modifications and extensions of these activities. The activities were designed so that instructors could select among the five activities as needed for their courses. The introductory videos in Activity 1 (https://www.stonybrook.edu/commcms/ecoevo/_publications/_downloads/sbeglia) could be used as review materials in majors courses, or as pre-course materials in a flipped classroom environment. Any of the activities could be used as pre-course assignments in preparation for more complex learning tasks; for example, the clicker questions from Activity 1 (Supporting File S1. Matter and Energy in Organisms – Activity 1 In-class slides) could be utilized as a pre-course quiz, and the model building activity would be useful as an optional or required take-home assignment.
SUPPORTING MATERIALS
- S1. Matter and Energy in Organisms – Activity 1 In-class slides
- S2. Matter and Energy in Organisms – Activity 2 In-class slides
- S3. Matter and Energy in Organisms – Activity 3 In-class slides
- S4. Matter and Energy in Organisms – Activity 4 Student worksheet (Plants conceptual model)
- S5. Matter and Energy in Organisms – Activity 4 Student worksheet (Animals conceptual model)
- S6. Matter and Energy in Organisms – Activity 4 In-class slides
- S7. Matter and Energy in Organisms – Activity 4 Rubric and examples
- S8. Matter and Energy in Organisms – Activity 5 In-class slides
- S9. Matter and Energy in Organisms – Activity 5 Student worksheet template
- S10. Matter and Energy in Organisms – Activity 5 Answer key
ACKNOWLEDGMENTS
We thank many colleagues at Michigan State University for producing the conceptual framework that guided our activities, most importantly the faculty and students involved in the BIODQC and the Carbon: Transformations in Matter and Energy (Carbon TIME) projects. We also thank Dr. John True for help with implementation of the lessons.
References
- Anderson CW, Sheldon TH, Dubay J. 1990. The effects of instruction on college nonmajors' conceptions of respiration and photosynthesis. J Res Sci Teach 27:761-776. doi:10.1002/tea.3660270806
- Wilson CD, Anderson CW, Heidemann M, Merrill JE, Merritt BW, Richmond G, Sibley DF, Parker JM. 2006. Assessing students' ability to trace matter in dynamic systems in cell biology. CBE Life Sci Educ 5:323-331. doi:10.1187/cbe.06-02-0142
- Mohan L, Chen J, Anderson CW. 2009. Developing a multi-year learning progression for carbon cycling in socio-ecological systems. J Res Sci Teach 46:675-698. doi:10.1002/tea.20314
- American Association for the Advancement of Science. 2011. Vision and change in undergraduate biology education: A call to action. American Association for the Advancement of Science, Washington D.C.
- NGSS Lead States. 2013. Next Generation Science Standards: For states, by states. National Academies Press, Washington D.C.
- Klemow K, Berkowitz A, Cid C, Middendorf G. 2019. Improving ecological education through a four-dimensional framework. Front Ecol Environ 17:71-71. doi:10.1002/fee.2013
- Doherty J, Ebert-May D, Pohlad BR. 2017. Ecology Learning Framework. CourseSource and Ecological Society of America. http://www.coursesource.org/courses/ecology
- Smith MK, Toth E, Borges K, Dastoor F, Johnson J, Jones EH, Nelson P, Page J, Pelletreau KN, Prentiss N, Roe JL, Staples J, Summers MM, Trenckman E, Vinson E. 2018. Using Place-Based Economically Relevant Organisms to Improve Student Understanding of the Roles of Carbon Dioxide, Sunlight, and Nutrients in Photosynthetic Organisms. https://doi.org/10.24918/cs.2018.1
- Darby BJ, Goodwin BJ. 2014. Using Pathway Maps to Link Concepts, Peer Review, Primary Literature Searches and Data Assessment in Large Enrollment Classes: An example from teaching ecosystem ecology. https://doi.org/10.24918/cs.2014.2
- Nehm RH. 2019. Biology education research: building integrative frameworks for teaching and learning about living systems. Discip Interdscip Sci Educ Res 1:15. doi:10.1186/s43031-019-0017-6
- Freidenreich HB, Duncan RG, Shea N. 2011. Exploring middle school students' understanding of three conceptual models in genetics. Int J Sci Educ 33:2323-2349. doi:10.1080/09500693.2010.536997
- Nehm RH, Ha M. 2011. Item feature effects in evolution assessment. J Res Sci Teach 48:237-256. doi:10.1002/tea.20400
- Kargbo DB, Hobbs ED, Erickson GL. 1980. Children's beliefs about inherited characteristics. J Biol Educ 14:137-146.
- Ha M, Nehm RH. 2014. Darwin's difficulties and students' struggles with trait loss: cognitive-historical parallelisms in evolutionary explanation. Sci & Educ 23:1051-1074. doi:10.1007/s11191-013-9626-1
- Maskiewicz AC, Griscom HP, Welch NT. 2012. Using targeted active-learning exercises and diagnostic question clusters to improve students' understanding of carbon cycling in ecosystems. CBE Life Sci Educ 11:58-67. doi:10.1187/cbe.11-02-0011
- D'Avanzo C, Anderson CW, Hartley LM, Pelaez N. 2012. A faculty-development model for transforming introductory biology and ecology courses. Bioscience, 62(4), 416-427. doi:10.1525/bio.2012.62.4.12
- Hartley LM, Wilke BJ, Schramm JW, D'Avanzo C, Anderson CW. 2011. College students' understanding of the carbon cycle: Contrasting principle-based and informal reasoning. Bioscience, 61(1), 65-75. doi:10.1525/bio.2011.61.1.12
- Anderson CW, de los Santos EX, Bodbyl S, Covitt BA, Edwards KD, Hancock JB, Lin Q, Morrison Thomas C, Penuel WR, Welch MM. 2018. Designing educational systems to support enactment of the Next Generation Science Standards. J Res Sci Teach 55:1026-1052. doi:10.1002/tea.21484
- Sbeglia G, Nehm R. 2019. Student reasoning about the movement of matter (MOM) across contexts: psychometric evaluation of cognitive coherence using Rasch analysis. Society for the Advancement of Biology Education Research (SABER). Minneapolis, MN.
- Gonzalez E, Rutkowski L. 2010. Principles of multiple matrix booklet designs and parameter recovery in large-scale assessments, p. 125-156. In von Davier, M, Hastedt, D (eds.), IERI Monograph Series: Issues and Methodologies in Large-Scale Assessments Volume 3.
- Tanner KD. 2013. Structure matters: twenty-one teaching strategies to promote student engagement and cultivate classroom equity. CBE Life Sci Educ 12:322-331. doi:10.1187/cbe.13-06-0115
- Papenberg M. 2019. minDiff: Minimize Differences Between Groups. R package version 0.01-3 (computer software).
- Boone WJ. 2016. Rasch analysis for instrument development: why, when, and how? CBE Life Sci Educ 15:rm4. doi:10.1187/cbe.16-04-0148
- Boone WJ, Staver JR, Yale MS. 2014. Rasch analysis in the human sciences. Springer, Dordrecht.
- Yang Y, He P, Liu X. 2018. Validation of an instrument for measuring students' understanding of interdisciplinary science in grades 4-8 over multiple semesters: a Rasch measurement study. Int J of Sci and Math Educ 16:639-654. doi:10.1007/s10763-017-9805-7
- Bond TG, Fox CM. 2001. Applying the Rasch model: Fundamental measurement in the human sciences. Lawrence Erlbaum Associates, Inc., Mahwah, NJ.
- Schwarz CV, Reiser BJ, Davis EA, Kenyon L, Achér A, Fortus D, Shwartz Y, Hug B, Krajcik J. 2009. Developing a learning progression for scientific modeling: Making scientific modeling accessible and meaningful for learners. J Res Sci Teach 46:632-654. doi:10.1002/tea.20311
Article Files
Login to access supporting documents
A Lesson on Matter and Energy at the Organismal Scale: Linking Patterns and Processes Across Diverse Taxa(PDF | 304 KB)
S1 Matter and Energy in Organisms-Activity 1 In-class slides.pptx(PPTX | 1 MB)
S2 Matter and Energy in Organisms-Activity 2 In-class slides.pptx(PPTX | 8 MB)
S3 Matter and Energy in Organisms-Activity 3 In-class slides_0.pptx(PPTX | 771 KB)
S4 Matter and Energy in Organisms-Activity 4 Student worksheet plants conceptual model.pdf(PDF | 173 KB)
S5 Matter and Energy in Organisms-Activity 4 Student worksheet animals conceptual model.pdf(PDF | 176 KB)
S6 Matter and Energy in Organisms-Activity 4 In-class slides.pptx(PPTX | 4 MB)
S7 Matter and Energy in Organisms-Activity 4 Rubric and examples.docx(DOCX | 368 KB)
S8 Matter and Energy in Organisms-Activity 5 In-class slides.pptx(PPTX | 140 KB)
S9 Matter and Energy in Organisms-Activity 5 Student worksheet template.docx(DOCX | 20 KB)
S10 Matter and Energy in Organisms-Activity 5 Answer key.docx(DOCX | 23 KB)
- License terms
Comments
Comments
There are no comments on this resource.