A Kinesthetic Modeling Activity to Teach PCR Fundamentals
Published online:
Abstract
Most molecular biology and biological sciences students understand that the polymerase chain reaction (PCR) is used to amplify DNA. However, we have found that some students experience conceptual misunderstandings, a lack of detailed comprehension of the PCR process, or difficulties with troubleshooting and predicting the effects of alterations to the standard PCR process. We hypothesized that a problem-based learning approach that incorporates a kinesthetic modeling of the PCR process could address these problems. During this hands-on learning activity, students “amplified” a specific region of template DNA through three cycles of PCR using a “toolkit” composed of a) intertwined, supercoiled, and double-stranded yarn representing template DNA, b) short wax sticks representing primers, and c) long wax sticks representing the PCR products. Instructors can introduce a variety of assessments, including real-time image capture of the models, pre- and post-activity assessment quizzes, and homework assignment to gauge student learning. We administered identical four-question quizzes worth 12 points to 28 undergraduate students before and after the activity. The mean score on the post-quiz was three points higher than the pre-quiz score, demonstrating a 75% increase in score. Moreover, we found that students who began the activity with lower levels of understanding experienced the most significant learning gains. This hands-on, student-centered, kinesthetic activity allowed students to (i) visualize PCR processes, (ii) construct a model of the PCR process, (iii) correct common misconceptions and sources of confusion, and (iv) actively engage in the learning process.
Citation
Haydel, S.E. and Stout, V. 2015. A Kinesthetic Modeling Activity to Teach PCR Fundamentals. CourseSource. https://doi.org/10.24918/cs.2015.8Society Learning Goals
Genetics
- Methods & Tools in Genetics
- What experimental methods are commonly used to analyze gene structure and gene expression?
Science Process Skills
- Process of Science
- Interpret, evaluate, and draw conclusions from data
- Construct explanations and make evidence-based arguments about the natural world
- Modeling/ Developing and Using Models
- Build and evaluate models of biological systems
- Communication and Collaboration
- Share ideas, data, and findings with others clearly and accurately
Lesson Learning Goals
Students will understand the fundamental processes that occur during initial PCR cycles.Lesson Learning Objectives
Students will be able to:- Draw or model the first three cycles of PCR, including the correct directionality (5’- and 3’-ends) of the primers and single-stranded PCR products.
- Diagram how single-stranded products from the first cycle of PCR are used as templates for subsequent PCR cycles.
- Demonstrate which parts of the primers will anneal to the original DNA template and subsequent PCR products.
- Model and demonstrate when the primer restriction enzyme sites are incorporated into double-stranded PCR products.
- Calculate the number of desired-length PCR products and long PCR products for each amplification cycle.
- Demonstrate how the incorporation of primer restriction enzyme sites into PCR products is a useful tool for subsequent cloning of the product into a vector.
Article Context
Course
Article Type
Course Level
Bloom's Cognitive Level
Vision and Change Core Competencies
Vision and Change Core Concepts
Class Type
Class Size
Audience
Lesson Length
Pedagogical Approaches
Principles of How People Learn
INTRODUCTION
In general, the majority of life sciences students understand that the polymerase chain reaction (PCR) method is used to amplify specific regions of DNA. However, we have found that many of our students harbor existing misconceptions or confusion about PCR and lack detailed understanding of the individual steps of the process (discussed below). Since it is difficult for instructors to implement conceptual change of misinformation or misconceptions by verbally communicating the information in a traditional lecture (1, 2), we aimed to address potential sources of confusion by implementing a kinesthetic modeling of the PCR process. This activity allowed students to kinesthetically generate a visual layout of the first three cycles of PCR and focus on fundamental processes that occur during initial phases of the amplification reaction.
Recently, the pedagogical importance of visual literacy and visualization in the education of biochemists and molecular biologists has been emphasized (3, 4) . While molecular visualization tools and animations enhance student understanding of molecular processes (5-7) , physical models of molecular or microscopic structures and concepts may improve understanding for many learners. For example, introductory biochemistry students made significant learning gains with repeated use of visual imagery and physical molecular models and reported that the physical models were most helpful in facilitating their learning (8). Moreover, introductory cell biology laboratory undergraduate students who used hand-held, physical models and molecular imaging programs during the semester responded with higher quality answers to specific higher-order cognitive thinking questions than students who only used computer imaging programs (9).
The hands-on, in-class PCR activity that we describe in this paper incorporates both visual and kinesthetic modes of learning, encourages students to recognize common mistakes, and clarifies existing knowledge, while emphasizing active engagement in understanding basic PCR processes. We anticipate that this kinesthetic activity would complement written learning exercises previously demonstrated to aid in student understanding of key PCR concepts, especially when combined with laboratory exercises (10). Although the idea of learning styles is debatable and perceived learning styles do not predict success, students do have preferences regarding how they learn (11, 12). We expect that this PCR modeling lesson would benefit students whose favored sensory learning modes are visual and kinesthetic, while still aiding students who prefer reading/writing and auditory learning modalities (13-16) .
This kinesthetic activity also allowed us to identify and address common PCR misconceptions and sources of student confusion. PCR processes that we have found confused our undergraduate students include the following examples:
(I) Related to DNA templates, students initially do not appreciate the impact of the use of PCR products as templates in subsequent reaction cycles. Moreover, during each cycle, students have difficulty visualizing and configuring the primers to anneal at the proper locations and in antiparallel configurations on different strands of the template DNA.
(II) For molecular cloning of PCR products, primers often have a 5’-end restriction enzyme sequence to aid in inserting PCR products into cloning vectors. In this case, students often do not realize that only the template-specific primer sequence, not the restriction enzyme sequence, of the primer should anneal to the original template DNA, thus affecting the calculations of melting temperatures of the primers. Additionally, there is often confusion associated with when and how the primers, including the restriction enzyme sequences, are incorporated into the desired PCR product.
(III) Regarding DNA extension, most students do not comprehend that the polymerase continues on the original template DNA leading to the generation of longer PCR products that are the predominant forms in the early cycles. Instead, students will draw incorrect diagrams whereby DNA extension on the original template DNA (e.g., chromosomal DNA) will inexplicably stop at the location of the second primer. At the end of 25-30 cycles, students often overlook that the longer products generated in the early cycles will be present in extremely low concentration compared to the desired amplified product.
(IV) Despite the possibility that students have been previously introduced to the general mathematical formula for determining the number of DNA copies produced per PCR reaction cycle (i.e., N X 2n, where N equals the number of initial DNA target molecules and n equals the number of cycles), this formula does not account for predicting the number of single-stranded products (or double-stranded products) that are the desired length. Therefore, students have difficulty determining the number of desired length and long, single-stranded PCR products that will result after each cycle.
Intended Audience
The intended audiences for this activity are life science majors and any other students who require an understanding of PCR fundamentals. This active learning module is suitable for any college-level course (lower or upper division) and can be modified to suit the specific needs of the class. We conducted the activity in a class entitled Techniques in Molecular Biology and Genetics, which is an upper division class primarily composed of juniors and seniors who were majoring in either microbiology or molecular biosciences and biotechnology.
Learning Time
This lesson can be structured to fit within a single class period of 50 – 75 minutes, depending on the needs of the instructor and students. The lesson described here requires 5 minutes to introduce the activity and the PCR “toolkit” (described below), 30 – 35 minutes for modeling activities, discussions, and explanations, and subsequent time to discuss and conclude the exercise, collect the materials, and present any future assessments (homework or in-class). Table 1, displayed at the beginning of the LESSON PLAN section, contains a teaching timeline.
Pre-requisite Student Knowledge
Knowledge of the basic principles of PCR is essential for successfully implementing this classroom exercise. Although students may indicate that they have previously learned about PCR, it is necessary to review basic PCR processes and steps.
SCIENTIFIC TEACHING THEMES
Active Learning
Activities outside of class: Textbook reading about PCR and videos that animate PCR processes (example: http://www.dnatube.com/video/2334/Polymerase-Chain-Reaction-PCR)
Activities in class: Modeling activity within small groups (3 students) with classroom-wide discussion occurring after student groups complete each PCR cycle.
Assessments
Pre-activity assessments: Quiz with multiple choice or short answer questions.
Post-activity assessments: Students take pictures of each modeled PCR cycle and of the completed activity and email the images to the instructor (or submit via course website) for evaluation; quiz with multiple choice or short answer questions; homework assignment to calculate and describe newly-synthesized DNA products during each PCR cycle; draw and label a diagram of PCR processes which include proper DNA polarity, primer annealing and directionality, generation of long and desired-length DNA products, incorporation of restriction enzyme sequences into the PCR product, determination of PCR product size, etc.
Inclusive Teaching
- The PCR modeling activity incorporates two major sensory modes of learning: visual and kinesthetic. This lesson leverages learning diversity in the classroom by accommodating visual learners who prefer drawings, pictures, and images and kinesthetic learners who appreciate physical involvement and activities, manipulation of objects, and experiences that emphasize doing (13, 14).
- Students who are Accommodators (perceived individual learning style that focuses upon concrete experiences and active experimentation) and prefer to learn through direct, hands-on experiences (15, 16) could benefit from this modeling activity.
- Small groups of three students ensure individual accountability and enable all students to actively engage in the exercise.
- Even students who perceive that they “know” PCR could benefit from understanding the theory behind PCR lab experiments.
LESSON PLAN
This lesson can be structured to fit within a single class period of 50 – 75 minutes, depending on the needs of the instructor and students. We presented and administered the pre-activity assessment quiz, introduced the lesson, performed the activity, actively discussed PCR fundamental processes, and introduced future assessments during a 50-minute class period. Table 1 contains a teaching timeline. We taught this activity in a traditional classroom with six rows of tables with each row seating eight or nine students. Performing this activity in a classroom with ample table space for the activity and room for instructors to interact with student groups is advantageous.

Table 1. Teaching PCR-Teaching Timeline
Before Class
Prior to beginning this active learning exercise, instructors should introduce the details of PCR. Alternatively (or additionally), the students should be given a reading assignment and shown an animation that sufficiently discusses and reviews the PCR process. When reviewing this process in class, instructors should emphasize the importance of primer specificity and orientation, the use of modified primers, DNA polymerase processivity, and the exponential increase of resulting DNA products due to the products of one cycle serving as templates in all subsequent cycles.
Materials
- Multi-colored 4-ply, 100 weight acrylic yarn, representing chromosomal DNA, with a section marked with black stripes to represent the DNA fragment for amplification (Figure 1A). The black stripes are spaced approximately 1 inch apart with marked sections extending for 1.5 to 3 feet of uncoiled, double-stranded yarn (Figure 3). The yarn may be reused by allowing the individual strands to twist together again, however, it is difficult to get the yarn strands to intertwine again perfectly. Each length of yarn is approximately 20 feet. Thus, each group of students will need 40 feet of yarn. Shorter pieces of yarn could be used if the instructor wants the students to separate or “denature” the entire supercoiled mass. Incorporation of shorter yarn strands would reduce instructor preparation time and would be useful in avoiding the potential misconception that only the DNA region for amplification will be denatured.
- Bendaroos® (readily available for purchase online or at retail stores) flexible building sticks (string coated with wax) (Figure 1B) to generate primers (Figure 1C) and DNA amplification products (Figure 2). Each group of students needs seven forward primers and seven reverse primers.
- Three sheets of colored Bendaroos® flexible building sticks. Each sheet has 42 pieces of 6-inch waxed-coated string (Figure 1B). While colored pipe cleaners could alternatively be used to generate primers and DNA amplification products, the waxed string easily sticks to the table surface, thus ensuring that the models stay properly aligned throughout the course of the activity.
- PCR “toolkit” – 6” x 9” manila envelope to hold the collection of a) seven forward primers, b) seven reverse primers, c) one copy of double-stranded, chromosomal template DNA, d) three sheets of different colored, flexible wax sticks (42 strands/sheet), e) clear tape, and f) scissors. One pair of scissors could be shared among two or three groups. Contents of the PCR “toolkit” are re-usable.

Figure 1. Materials needed for administering the kinesthetic PCR modeling activity. (A) Circular, supercoiled, double-stranded chromosomal DNA, represented by multi-colored yarn, that serves as template DNA in the PCR reaction. Black stripes (white arrows) mark the DNA region intended for amplification. (B) Single sheet of the Bendaroos® colored wax sticks. Three sheets (different colors) of flexible, wax-coated string were used to generate DNA amplification products in three cycles of PCR. Additional colors were used to create primers. (C) Bendaroo® primers with the longer 2-inch blue or orange segments representing the DNA template-binding region and the shorter 0.5-inch yellow segment representing the 5’-end restriction enzyme site. Two different colored Bendaroo® strings were pressed together and molded to form the DNA primers. To create the primers, we recommend using Bendaroos® wax sticks that are different colors than the three colors used to represent DNA extension products.

Figure 2. DNA denaturation and the first three rounds of PCR. (A) The region of the DNA template for amplification (yarn marked with black stripes) is shown after the supercoiled, double-stranded DNA template was denatured to separate the DNA strands. (B) PCR cycle number 1 is shown with annealing of forward (red) and reverse (green) primers, each with a restriction enzyme site at the 5’ end, represented by short yellow wax sticks. he two single-stranded PCR extension products are represented by the orange wax sticks. The red and green wax sticks anneal specifically to the DNA template, while the yellow wax restriction enzyme site does not anneal. (C) Similar to the first cycle, PCR cycle number 2 is shown with the annealing of forward and reverse primers to all possible templates and four single-stranded PCR extension products (shown as blue wax sticks). The two single-stranded PCR extension products from PCR cycle number 1, shown as orange wax sticks, are also used as DNA templates for PCR cycle number 2. (D) Similar to the first two cycles, PCR cycle number 3 is shown with the annealing of forward and reverse primers and eight single-stranded PCR extension products (shown as red wax sticks). The single-stranded PCR extension products from PCR cycles 1 and 2, created using orange and blue wax sticks, respectively, are also used as DNA templates for PCR cycle number 3.
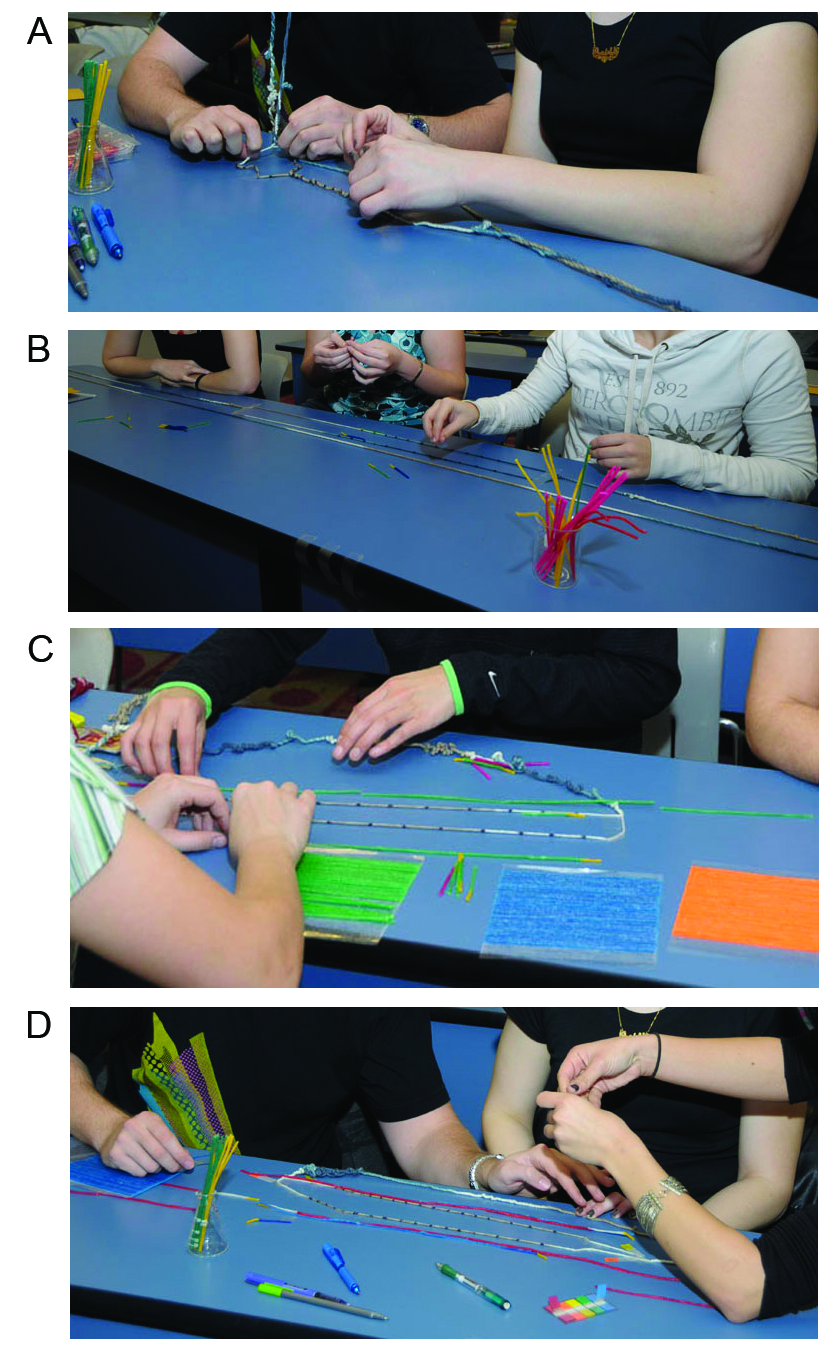
Figure 3. Pictures of students engaged in the kinesthetic PCR modeling processes. (A) chromosomal DNA denaturation; (B) primer annealing; (C) DNA extension; and (D) PCR cycle 2.
Instructor Preparation
Prior to beginning this active learning exercise, we assigned textbook readings to introduce details of PCR and instructed students to watch animations reviewing PCR processes. There are many videos that show animations of PCR, but three that we found valuable are listed below:
1) http://www.dnatube.com/video/2334/Polymerase-Chain-Reaction-PCR (DNA Tube Scientific Video Site)
2) http://www.youtube.com/watch?v=eEcy9k_KsDI&feature=related (Garland Science)
3) http://www.youtube.com/watch?v=2KoLnIwoZKU&feature=related (DNA Learning Center)
Likewise, there are many websites that describe PCR:
1) For general information about PCR:
- http://www.ncbi.nlm.nih.gov/probe/docs/techpcr/
- http://en.wikipedia.org/wiki/Polymerase_chain_reaction
2) For specific information about adding a restriction enzyme site to the ends of the primers:
- http://www.addgene.org/plasmid-protocols/pcr-cloning/
- http://oxfordgenetics.com/cloning-resources/cloning-guides/adding-restriction-sites-by-pcr
To address common PCR misconceptions and sources of student confusion, we emphasized the importance of primer specificity and orientation, the use of modified primers, DNA polymerase processivity, and the exponential increase of resulting DNA products due to the use of products as templates in subsequent cycles.
Supercoiled, chromosomal template DNA was created using multi-colored yarn (Figure 1A). Multi-colored yarn was chosen to show the vast number of “different” genes in a chromosome. A demonstration video showing how multi-colored yarn was used to model supercoiled, chromosomal DNA is shown in Supplemental File S1. Cut two, identical 20 ft pieces of multi-colored yarn. Align the colors of the two pieces of yarn, and join the two strands with a knot at one end. Secure the knotted end with tape to an immobile object. Then, intertwine the two yarn strands together to generate a yarn model of double-stranded chromosomal DNA. Although one may manually intertwine the yarn, the process is very time-consuming. To expedite the process, we used an electric drill equipped with a thinset and grout mixer paddle to facilitate the intertwining. The unsecured ends of the two pieces of yarn were tied to the outer edges of the drill mixer paddle. Intertwining was initiated and continued using the electric drill with intermittent manual alignment of the respective colors (i.e., aligning dark blue sections to represent a “gene”) on each piece of yarn. Repeat the cycles of intertwining and aligning until the strands of yarn are difficult to manually separate. Upon completion of the intertwining and color aligning process, we removed the yarn strands from the drill mixer paddle and joined the two strands together with a knot. We subsequently tied the two knotted ends together and allowed the mass to spontaneously supercoil. We found that it was important to not let the intertwined yarn uncoil before tying the knotted ends together. Once the ends were tied together, the tightly intertwined yarn formed a large, double-stranded supercoiled mass (Figure 1A), reminiscent of the genomic DNA complexity that occurs inside bacterial cells. Shorter pieces of yarn could be used if the instructor wants the students to separate or “denature” the entire supercoiled mass. Incorporation of shorter yarn strands would reduce instructor preparation time and would be useful in avoiding the potential misconception that only the DNA region for amplification will be denatured.
To create the DNA primers (Figure 1C), we used Bendaroos® flexible building sticks (Figure 1B). We cut the wax sticks (a single color) into thirds (~ 2 inches) to represent the DNA-specific primer sequence. Each PCR kit will contain seven of these primers. We chose a differently colored wax stick and cut ~1/2-inch fragments to represent restriction enzyme sites (at the 5’ end of the primer). To generate a single full-length, modified primer, the ends of one short and one long fragment were pressed and sealed together (just with the physical pressure and heat from our fingers) to generate a two-toned Bendaroo. The forward and reverse primers were represented by using different colored wax sticks (Figure 1C). For three PCR cycles, the PCR kit contained seven forward and seven reverse primers.
A section of the double-stranded DNA that each student group was to “amplify” was chosen at random, and the yarn fragment was striped using a permanent marker (Figure 2A). The length of the DNA regions to be amplified was varied for the different student groups, ranging from 18 to 36 inches.
Instructor Instructions
1) Deliver the learning objectives and present the activity using a PowerPoint presentation (Supplemental File S2). Tell students that they will perform this activity in groups of three students. Alternatively, in small classes, students could perform this activity individually. In very large classes or when the instructor is limited to 10-15 minutes, an entire class, interactive demonstration with discussion may be warranted.
2) We presented our students with the following scenario: “Each self-selected group of three students has been assigned a gene to amplify from the chromosome of Whodatamonas saintsatium, a newly discovered (obviously fictitious) bacterium that helps grown men win football games on Sunday. (The first author is a New Orleans native and lifelong Saints fan.) We have isolated ‘chromosomal DNA’ for you because we are such wonderful instructors.”
3) Give each group of students their PCR “toolkit” with the necessary reagents needed to amplify a specific DNA fragment. Each PCR toolkit consists of an envelope of “reagents” which includes
a) an “aliquot” (one copy) of the chromosomal DNA (multi-colored, supercoiled yarn) with the DNA region intended for amplification marked with black stripes (Figure 1A).
b) multiple copies of forward and reverse primers (each primer has been modified with a restriction enzyme site shown in yellow in Figure 1C). Note: Instructors could inform students that, typically, there are thousands of forward and reverse primers available for each template molecule.
c) three sets of differently colored Bendaroos® flexible building sticks (Figure 1B) with one color representing extension products for each PCR cycle.
4) Instructors should indicate that there is a single, supercoiled template in the PCR “toolkit”, whereas typically, PCR reactions contain multiple templates. In addition, instructors should emphasize that the supercoiled yarn is double-stranded (Figure 1A). Therefore, students must “denature” and separate the strands of yarn (Figure 2A). Students should be aware that during an actual PCR reaction, the template DNA is completely denatured to single-stranded DNA during the initial and subsequent denaturation steps. During this exercise, students will not fully denature the template DNA to generate single-stranded DNA. Alternatively, students will locate the black-striped region of the template DNA, representing the specific region for amplification, and separate (“denature”) this region only (Fig. 2A). After locating all of the black marks and separating the two strands of yarn, students should tape the individual yarn strands to the desk to avoid “renaturation” of the DNA (Fig. 2A).
5) Instruct each group that they will proceed through the first three rounds of a PCR cycle using the supplied primers and the colored wax-coated strings to represent the PCR extension products (Figure 2). The students can overlap, join, or cut the waxed strings as necessary. The waxed strings are bendable and easily stick to hard surfaces. Instructors should note that the students’ hands serve as the thermostable polymerase to facilitate DNA synthesis and that “dNTPs” are available in abundance (although not supplied in the reagent toolkit). Alternatively, the instructor may query the students regarding which essential components of the PCR reaction are not supplied in the reagent toolkit and the “enzymatic function” facilitated by their hands. Instructors may vary the number of rounds of the PCR cycle as necessary, remembering that additional primer sets will be needed for additional rounds and that organization of the PCR products can get confusing with additional rounds (Figure 3).
6) Groups should complete one PCR cycle, then wait until all of the other groups have also completed one cycle. Once all groups have “completed” the first PCR cycle, allow different groups to discuss their approach, thought processes, and results. Once a clear understanding of this PCR cycle is established, proceed to the next cycle and subsequent discussion, and then to the third cycle. Some questions for instructors to consider during in-class circulation among the student groups and during the discussions:
a) Are the restriction enzyme sites within the primers annealing to the chromosomal DNA? The restriction enzyme site should not anneal to the chromosomal DNA. Students should bend the primer restriction enzyme site away from the chromosomal DNA.
b) How far did the first single-strand products extend? Did extension miraculously stop at the end of the other primer? DNA polymerase continues until the temperature change in the next cycle denatures the double-stranded DNA formed by the newly synthesized strand and the template strand. Alternatively, DNA polymerase stops when it comes to the end of template.
c) Are the primers located in the correct place on the chromosome? Are the forward and reverse primers displayed in the correct orientation? The location of the restriction enzyme site within the primer gives the students an easy reference for locating the 5- end of the primer and helps with maintaining proper DNA polarity. The primers should be pointing “toward” one another with the DNA region to be amplified located in between the primers.
d) What happens to the products generated in previous cycles? They are used as additional templates for subsequent cycles. Thus, you begin with one copy of the DNA, present within the chromosomal template, and end up with three copies of the DNA, one present within the chromosomal template and two PCR fragments.
e) How many new double-stranded DNA products are generated after each cycle?
f) During the second amplification cycle, are the 5’-end restriction enzyme sites of the primers annealing correctly to long extension products generated in cycle 1?
g) Do the students realize that the long extension products will be in extremely low concentrations compared to the desired amplified product (after 25-30 cycles)? These long products will only be produced by the original chromosomal DNA template, so you will only get two with each cycle At the end of 30 cycles, you would have only 60 copies of these long DNA products and XX copies of the actual target product.
Student Instructions
Instructors may provide the students with a verbal introduction to the exercise (5-10 minutes) or by generating written guidelines that are handed out to the student groups.
Today, you will use a PCR “toolkit” to complete a hands-on simulation of PCR. The goal of the activity is to amplify the designated region of DNA on the chromosome (marked with black stripes) such that a restriction enzyme site is present on both ends of the resulting amplification products. All DNA products must be properly aligned with the template DNA, and you must ensure that the correct antiparallel configuration of the amplified DNA and primers is maintained. Each group will be required to demonstrate and explain the results of each cycle before proceeding to the next cycle.
1) Check out the PCR “toolkit”: Working in your group, use the provided PCR “toolkit” (envelope of materials representing the PCR “reagents”) to simulate the first three rounds of a PCR cycle. The PCR “toolkit” or envelope contains a pair of scissors and the following “reagents”:
(a) chromosomal DNA – one DNA double helix represented by two pieces of multi-colored yarn that are tightly intertwined (“double-stranded”). The gene is marked on both strands with black stripes, indicating the region that must be amplified. Bacterial DNA is circular, so there are no “free” 3’- or 5’-ends. Each strand of DNA in a eukaryotic chromosome has a free 5’- and 3’-end.
(b) primers – seven copies of each of two differently-colored short pieces of waxed string. Each primer has a long section (about 2 inches) that represents the DNA-specific primer sequence and a shorter section (about 1/2 inch) that represents a 5’-end restriction enzyme sequence.
(c) DNA extension products – long pieces of waxed string with a single color to be cut or joined together to represent the extension products synthesized by heat-stable DNA polymerase during each PCR cycle.
2) Denature the DNA: The supercoiled yarn is double-stranded (Figure 1A) and therefore, you must “denature” or separate the strands of yarn (Figure 2A). During an actual PCR reaction, the template DNA is completely denatured to single-stranded DNA during each denaturation step. However, during this simulation, you will not fully denature the template “DNA” to generate single-stranded DNA. Instead, locate the black-striped region of the template DNA, representing the specific region for amplification, and separate (“denature”) this region only (Figure 2A). Once you have separated the two strands of yarn, tape the individual yarn strands to the desk to avoid premature “renaturation” of the template DNA (Figure 2A).
3) Synthesize the PCR products for Cycle 1: Use the supplied primers and the colored wax-coated strings to generate the PCR extension products (Figure 2B-D). You should overlap, join, or cut the waxed strings as necessary to represent the PCR product that is synthesized by DNA polymerase during the extension phase of the PCR cycle. The waxed strings are bendable and easily stick to hard surfaces.
4) Examine the products: Stop after your group has completed the DNA synthesis (extension) phase of Cycle 2. Designate a group member to explain your results and make sure that this spokesperson can explain everything. Once all of the groups have “completed” this PCR cycle (Figure 2B-D), one or more groups will explain their approach, thought processes, and results to the rest of the class.
5) Repeat Steps 3 and 4 to simulate Cycle 2.
6) Repeat Steps 3 and 4 to simulate Cycle 3.
Student Assessments
With this kinesthetic modeling activity and series of lessons/discussions related to fundamental PCR processes, instructors can choose to assess student learning several ways. One real-time, formative assessment strategy includes having each group use their cell phones or other devices to take pictures of their PCR models after each cycle and upon activity completion (i.e., completion of Cycle 3). These pictures could be easily emailed to the instructor during class, uploaded via the course website, or shared using course sharing software. A second assessment strategy includes identical pre- and post-activity assessment quizzes which could be administered prior to initiating the exercise and days or weeks after completing the activity (Table 2). The quiz, composed of four questions, was designed to address some common misconceptions and sources of confusion we noted in our students (described above). The ideal answers with associated point values are shown in Table 2. As a third assessment, instructors could give a homework assignment after the activity to help students assess their own learning. An example homework assignment consists of completing a table (Supplemental File S3, with correct answers in parentheses) in which students analyze the results of the active learning exercise and calculating the number of various PCR products that would result after consecutive PCR cycles. The analytical homework exercise will encourage students to draw out the products generated during PCR cycles and/or review the images of their generated models, thus practicing the process they learned in class. A fourth assessment strategy includes in-class or homework worksheets that require students to locate primer annealing sites in a given DNA template, predict PCR product sequences and sizes, identify restriction enzyme recognition sites, and analyze primer polarity (Supplemental Files S4, S5, and S6). Instead of grading the assignments, instructors could choose to informally discuss the homework answers, thus allowing instructors to gauge student progression toward achieving the learning objectives. However, instructors may choose to use the homework assignment as a summative assessment by collecting student work and assigning it a grade that counts toward the student’s course grade.

Tables 2 and 3. Teaching PCR
TEACHING DISCUSSION
For this interactive lesson, we assessed student achievement of the learning objectives with written quizzes, formative assessments, and ongoing descriptive feedback during the class period. Students remained very engaged and on task for the entire active learning exercise. They seemed to enjoy figuring out how to construct their models and many groups were able to self-correct problems that arose. However, a few student groups (two to three out of 10) struggled with mastering the first learning objective, i.e. assembling and modeling the process of PCR and maintaining the correct orientation of the primers and templates. With our ongoing guidance throughout the activity, we observed that the performance of these groups improved while performing cycles two and three. Thus, through the use of formative assessments during the interactive instructional process, we were able to focus the students on the learning objectives. It was a very enjoyable activity for us. We appreciated being able to assist struggling students and then subsequently observe them mastering the objectives. It was much easier to observe and address problems and misconceptions about the process in real time as we circulated among the groups.
Although instructor preparation of the first series of PCR “toolkit” sets takes several hours, most of the contents can be re-used. Upon completion of the activity, some of the Bendaroos that students cut to fit their models could be discarded. Additionally, the supercoiled yarn does not rewind itself well after student “denaturation”, so it may be advantageous to generate new supercoiled yarn samples.
This lesson was presented in an upper division lecture course, entitled Techniques in Molecular Biology and Genetics, which was primarily composed of juniors and seniors who were majoring in either microbiology or molecular biosciences and biotechnology. Twenty-eight students participated in the activity and both the pre- and post-activity assessments (Table 2) (exempt status by the ASU Institutional Review Board; IRB ID 00001352). On the day of this activity, we began with a short (5 minute) lecture focused upon the components of a PCR reaction, followed by approximately 10 minutes to distribute, administer, and collect the pre-assessment quizzes. After students completed the pre-activity assessment quiz, instructions for the activity were given both verbally and in written form (on a PowerPoint slide) (Supplement File S2). Students performed the activity in self-selected groups of three for approximately 30 minutes (Figure 3). After each “cycle” of the activity, one student group volunteered to discuss and explain their thought processes and approaches for completing the exercise. These continuing discussions, combined with ongoing instructional assistance throughout the activity, provided evidence of student learning, intellectual engagement, and higher order cognitive thinking skills. During the last 5 minutes of class, the activity concluded and homework instructions were given. Five days later, during the next class period, the identical assessment quiz was re-administered during the first 10 minutes of class. It is important to note that the students did not have prior knowledge of the assessment quizzes or the specifics of the active learning exercise, and they did not get feedback from the pre-activity assessment quiz before they took the post-activity assessment quiz.
To assess student learning and gauge student progress, we compared the scores on the pre- and post-activity assessment quizzes (Table 3) and evaluated the results. The average increase of 2.95 points, comparing the pre- and post-assessment scores, was statistically significant (p < 0.0001) (Table 3). Mean quiz scores increased from 4.05 on the pre-activity quiz to 7.00 on the post-activity quiz (Table 3). When the pre-assessment total scores were sorted and divided into three groups and compared to the post-assessment scores, we found that the lowest third and middle third of the class improved the most, with an average increase of 3.94 and 3.55 total points, respectively, while the upper third of the class improved the least (1.28 points). Overall, these results indicated that students corrected some of their previously held misconceptions and achieved deeper understanding of PCR details. Moreover, students who began the activity with greater levels of misunderstanding attained the greatest learning gains.
The average increase of 0.23 points for question 1 (Table 2) on the assessment was not statistically significant (p = 0.3196), allowing us to conclude that the activity and discussion did not establish the importance of specific primer-template annealing in the early cycles as a mechanism to reduce the amplification of non-specific PCR products. Assessment results of question 2 (Table 2) revealed that students learned that the primers, including the restriction enzyme site, were incorporated into the PCR products. For question 2, the comparative averages of the pre- and post-activity assessment quizzes increased by 0.79 points, which was statistically significant (p = 0.0006) (Table 3). For question 3, the post-assessment average increase was 1.4 points (p < 0.0001) (Table 3), thus revealing that students gained an understanding that long extension products result when primers anneal to the original template DNA. Based on the scores from question 4, students’ ability to predict the number of single-stranded PCR products that result after each PCR cycle improved (average increase of 0.54 points, which was statistically significant; p = 0.0253), although not as significantly as with questions 2 and 3 (Table 3).
After administering the pre- and post-assessments, we recognized that question 4 was ambiguous (Table 2). The intent of this question was for students to indicate the total number of newly synthesized single-stranded DNA products resulting after the first two rounds of PCR, although we did not use the word “new” in the question. Since cycles 1 and 2 yield two and four new single-stranded products, respectively, a total of six new, single-stranded products are synthesized after two PCR cycles (Table 2). However, some students also included the two template strands in their calculations. Because students had correctly calculated the six new, single-stranded PCR products (in addition to the two original template strands), these students were given partial credit (1.5 points). A few other students indicated that there were no single-stranded products at the end of the PCR cycle because all newly synthesized strands were annealed to their template strands. Because this answer was true, students who responded with “zero” and provided an explanation received full credit (3 points) for question 4. Although we did not indicate on the quiz that students should “defend” their answer for question 4, full credit was given if their reasoning and critical thinking was correct. Students who responded “zero”, but did not defend their answer received 0 points. We suggest that instructors modify question 4 to address these issues and to encourage students to scientifically defend their answers. While we acknowledge that this kinesthetic activity did not enhance the students’ ability to accurately answer question 1 (Table 2), the overall improved student gains on the post-assessment quizzes revealed that the exercise was effective and that student learning was significantly enhanced.
In addition to the potential modifications to the pre- and post-activity assessment quizzes discussed above, this kinesthetic learning activity could be additionally modified in several ways to address the specific needs of the student audience.
(1) To highlight primer specificity, an instructor could color coordinate the specific yarn segment (template DNA) with a color-matching flexible, waxed string (primer). In this way, students would search the entire multi-colored yarn mass searching for the colored region that matches the small, flexible wax string. Upon locating the matching color segments, the primer wax string would “specifically” anneal to the colored DNA segment based on color similarity. This modification would enable students to visualize the processes that occur in a PCR mixture whereby the primer specifically anneals to the template DNA based on sequence similarity.
(2) Since the addition of a 5’-end restriction enzyme sequence is considered a primer modification, one could choose to use primers that are specific to the template DNA and lack the 5’-end modification. The exercise could then relate to the use of PCR as a diagnostic tool or in forensics applications.
(3) Additional assessment exercises that were not included in the original activity are shown in Supplemental File S4. Example worksheets to assess achievement of learning objectives 3, 4, and 6 are included as Supplemental File S5 (answer key included as Supplemental File S6).
(4) For an upper division molecular genetics course, instructors could modify the exercise to introduce primer point mutations. As a result, students could visualize the use of PCR as a molecular tool to introduce mutations into an amplified DNA sequence.
SUPPLEMENTAL MATERIALS
- Table 1. Teaching PCR-Teaching timeline
- Table 2: Teaching PCR-Pre- and post-activity assessment quiz questions and answers with assigned point values added in parentheses.
- Table 3: Teaching PCR-Comparison of student scores on the pre- and post-activity assessment quizzes.
- Figure 1: Teaching PCR-Materials needed for administering the kinesthetic PCR modeling activity.
- Figure 2: Teaching PCR-DNA denaturation and the first three rounds of PCR
- Figure 3: Teaching PCR-Pictures of students engaged in the kinesthetic PCR modeling activity.
- Supplemental File S1: Teaching PCR-Demonstration video showing how multi-colored yarn was used to model supercoiled, chromosomal DNA.
- Supplemental File S2: Teaching PCR-PowerPoint presentation to deliver the learning objectives and present the activity.
- Supplemental File S3: Teaching PCR-Example of a homework assignment (with answers shown in parentheses) that could be given after students complete the PCR modeling lesson.
- Supplemental File S4. Teaching PCR-Additional assessment exercises that were not included in the original activity
- Supplemental File S5. Teaching PCR-Example worksheets to assess achievement of learning objectives 3, 4, and 6
- Supplemental File S6. Teaching PCR-Answer key for the example worksheets to assess achievement of learning objectives 3, 4, and 6
ACKNOWLEDGEMENTS
This research was supported by an Undergraduate Education in Biology Award from the ASU College of Liberal Arts and Sciences in agreement with the National Academies – Howard Hughes Medical Institute Summer Institute on Undergraduate Education in Biology. We thank the students in MIC/MBB 445 Techniques in Molecular Biology and Genetics (ASU School of Life Sciences) for their flexibility and commitment to active learning, Kevin Coons for photography and videography, and Travia Steward for video editing.
References
- Macbeth D. 2000. On the actual apparatus for conceptual change. Sci. Educ. 84(2):228-264.
- Modell H, Michael J, Wenderoth MP. 2005. Helping the learner to learn: The role of uncovering misconceptions. Am. Biol. Teach. 67(1):20-26.
- Schonborn KJ, Anderson TR. 2006. The importance of visual literacy in the education of biochemists. Biochem. Mol. Biol. Educ. 34(2):94-102.
- McClean P, Johnson C, Rogers R, et al. 2005. Molecular and cellular biology animations: Development and impact on student learning. Cell Biol. Educ. 4(2):169-179.
- White B, Kim S, Sherman K, Weber N. 2002. Evaluation of molecular visualization software for teaching protein structure: differing outcomes from lecture and lab. Biochem. Mol. Biol. Educ. 30(2):130-136.
- Booth D, Bateman RCJ, Sirochman R, et al. 2005. Assessment of molecular construction in undergraduate biochemistry. J. Chem. Edu. 82(12):1854-1858.
- Wu H-K, Krajcik JS, Soloway E. 2001. Promoting understanding of chemical representations: students' use of a visualization tool in the classroom. J. Res. Sci. Teaching 38(7):821-842.
- Roberts JR, Hagedorn E, Dillenburg P, Patrick M, Herman T. 2005. Physical models enhance molecular three-dimensional literacy in an introductory biochemistry course. Biochem. Mol. Biol. Educ. 33(2):105-110.
- Harris MA, Peck RF, Colton S, et al. 2008. A combination of hand-held models and computer imaging programs helps students answer oral questions about molecular structure and function: A controlled investigation of student learning. CBE Life Sci. Educ. 8(1):29-43.
- Phillips AR, Robertson AL, Batzli J, Harris M, Miller S. 2008. Aligning goals, assessments, and activities: An approach to teaching PCR and gel electrophoresis. CBE Life Sci. Educ. 7(1):96-106.
- Riener C, Willingham D. 2010. The myth of learning styles. Change: The Magazine of Higher Learning 42(5):32-35.
- Pashler H, McDaniel M, Rohrer D, Bjork R. 2008. Learning styles: concepts and evidence. Psychological Science in the Public Interest 9(3):105-119.
- Fleming ND. VARK, A Guide to Learning Styles. http://vark-learn.com/
- Fleming ND, Mills C. 1992. Not another inventory, rather a catalyst for reflection. To Improve The Academy 11:137-155.
- Kolb DA. 1971. Individual learning styles and the learning process. MIT Press, Cambridge, MA.
- Smith D, Kolb DA. 1985. User guide for the learning-style inventory. McBer, Boston.
Article Files
Login to access supporting documents
A Kinesthetic Modeling Activity to Teach PCR Fundamentals(PDF | 1 MB)
Supplemental File S2. Teaching PCR-PowerPoint presentation to deliver the learning objectives and present the activity.pptx(PPTX | 547 KB)
Supplemental File S3. Teaching PCR-Example of a homework assignment with answers shown in parentheses that could be given after students complete the PCR modeling lesson.docx(DOCX | 20 KB)
Supplemental File S4. Teaching PCR-Additional assessment exercises that were not included in the original activity.docx(DOCX | 23 KB)
Supplemental File S5. Teaching PCR-Example worksheets to assess achievement of learning objectives 3 4 and 6.docx(DOCX | 46 KB)
Supplemental File S6. Teaching PCR-Answer key for the example worksheets to assess achievement of learning objectives 3 4 and 6.docx(DOCX | 49 KB)
- License terms
Comments
Comments
There are no comments on this resource.