Using Place-Based Economically Relevant Organisms to Improve Student Understanding of the Roles of Carbon Dioxide, Sunlight, and Nutrients in Photosynthetic Organisms
Published online:
Abstract
Biology students require broad preparation for diverse careers including agriculture, natural resource management, and laboratory research. Concurrent with this need, employers are seeking applicants who have the scientific skills that allow them to solve problems related to locally relevant economic systems and develop ways to foster economic growth. To support these efforts, biology faculty from six different campuses in the University of Maine System collaborated to develop an economically relevant activity where students differentiate the roles light energy, carbon dioxide, and nutrients have in photosynthetic organisms. In addition, the activity addresses the relationship between photosynthesis and global carbon dioxide cycles, as well as the potential impacts of rising global atmospheric carbon dioxide on economic industries that rely on these processes. The activity was taught in 11 classrooms throughout the University of Maine System, and student performance was assessed using a multiple-choice pre/post-test, pre/post constructed response questions, in-class clicker questions with peer discussion, and exam questions. Here we report that the activity improved student learning and that combining the expertise of University of Maine System faculty and the Hurricane Island Center for Science and Leadership provided the opportunity to integrate biological concepts with economic development. Although the examples in this lesson have economic relevance in the state of Maine, the examples can be modified to align with other regional economic systems.
Citation
Smith, M.K., Toth, E.S., Borges, K., Dastoor, F., Johnston, J., Jones, E.H., Nelson, P.R., Page, J., Pelletreau, K., Prentiss, N., Roe, J.L., Staples, J., Summers, M., Trenckmann, E., and Vinson, E. 2018. Using Place-Based Economically Relevant Organisms to Improve Student Understanding of the Roles of Carbon Dioxide, Sunlight, and Nutrients in Photosynthetic Organisms. CourseSource. https://doi.org/10.24918/cs.2018.1Society Learning Goals
Cell Biology
- Matter & Energy Transformation
- How do cells transform energy and cycle matter?
Ecology
- Matter and Energy in Ecosystems
- How does matter and energy move in an ecosystem?
- How do organisms obtain and use matter and energy to live and grow?
Plant Biology
- Organization of Matter and Energy Flow
- How do plants obtain and use matter and energy to live and grow?
- Plants in Ecosystems
- How do matter and energy move through an ecosystem?
Lesson Learning Goals
Students will understand the roles light energy, carbon dioxide, and nutrients have in photosynthetic organisms.Lesson Learning Objectives
At the end of this lesson, students will be able to:- Describe the roles of light energy and carbon dioxide in photosynthetic organisms.
- Identify the effect of nutrients on the growth of photosynthetic organisms.
- Describe global cycles in atmospheric carbon dioxide levels and how they relate to photosynthetic organisms.
Article Context
Course
Article Type
Course Level
Bloom's Cognitive Level
Vision and Change Core Competencies
Vision and Change Core Concepts
Class Type
Class Size
Audience
Lesson Length
Pedagogical Approaches
Principles of How People Learn
Assessment Type
INTRODUCTION
Learning about the roles of sunlight and carbon dioxide in photosynthetic organisms is necessary to understand global ecological processes. Notably, Pathways and Transformations of Energy and Matter is one of the five core concepts in Vision and Change (1) and Matter and Energy in Organisms and Ecosystems is one of the Next Generation Life Science Standards (2). This lesson focuses on the role of carbon dioxide as the primary contributor of biomass for both terrestrial and aquatic photosynthetic organisms, the conversion of light energy to chemical energy needed to incorporate carbon dioxide into biomass, and the effect of fertilizers on photosynthetic organism growth (rev in. https://www.nature.com/scitable/knowledge/library/energy-economics-in-ecosystems-13254442).
Without a solid understanding of these processes, confusion about global issues, such as the effects of increasing levels of atmospheric carbon dioxide, can occur. For example, most undergraduates surveyed at one large public institution correctly identified that carbon dioxide is a gas that comes from the burning of coal and oil, yet they often incorrectly concluded that elevated carbon dioxide levels are the cause of ozone depletion (3). They also had trouble identifying the causes of increased carbon dioxide levels beyond local pollution, and were surprised at how much their own everyday behaviors increased their carbon footprint.
Student conceptual difficulties about the role of sunlight and carbon dioxide in photosynthesis have been documented by multiple research studies. For example, college majors and non-majors from a variety of institutions in several countries are often confused about what provides energy for a plant (4,5,6). When students are asked, "Which of the following are energy sources for a plant?" and allowed to select any number of options from a list that includes sunlight, nutrients, fertilizer, soil, water, carbon dioxide, etc., students will often correctly answer sunlight, but also select one or more incorrect options. When students were questioned about their confidence in choosing an additional answer, such as soil, approximately half of the students indicated they were confident in their selection (6).
In addition, students often incorrectly explain the role of carbon dioxide in photosynthetic organisms. For example, some students think that carbon dioxide provides energy for photosynthesis (7), and do not understand that the absorption of carbon dioxide and release of oxygen increases the mass of the plant (5). One way to probe this conceptual difficulty is to ask students to explain where the biomass comes from when a seed grows into a tree. Fewer than 20% of incoming students in an introductory biology majors course at a large public institution correctly answered that carbon dioxide from the air was the source of this biomass (8). Instead, most incorrectly stated that the biomass comes from organic substances or minerals in the soil (8,9).
Notably, these conceptual difficulties stay with students throughout their entire undergraduate biology career. For example, when students from 34 different college and universities took an ecology and evolution assessment called Ecology and Evolution Measuring Achievement and Progression in Science (EcoEvo-MAPS), many of the most challenging questions for seniors were aligned with energy and matter themes (7). The most common conceptual difficulties included: carbon in the soil being directly incorporated into plant tissues, carbon dioxide providing the energy required for photosynthesis, and fertilizers providing both energy and nutrients.
There are several types of instructional activities that have been developed to address undergraduate student conceptual difficulties about the role of sunlight, carbon dioxide, and nutrients in photosynthetic organisms. These activities include concept mapping, where students can draw connections between carbon dioxide, photosynthesis, and sunlight (10), and repositories of clicker questions related to conceptual difficulties concerning energy and matter (http://biodqc.org/node/518). There are also free drawing assessment questions and rubrics that have been developed to help instructors diagnose the frequency of common student conceptual difficulties (11,12). In addition, there are worksheet and laboratory activities that allow students to explore and reproduce historical experiments (http://biodqc.org/node/326; 13). For example, students can learn about J.B. van Helmont's willow tree experiment where he showed that soil is not the primary source of plant biomass (14,15). Finally, there is a modeling activity for non-majors where students analyze data from measured atmospheric carbon dioxide levels (16).
Although several of these instructional activities target student learning about the role of sunlight, carbon dioxide, and nutrients in photosynthetic organisms, there is a need for classroom activities that address specific student conceptual difficulties (e.g., 5,6,8,9), provide instructors with timely feedback on student learning using multiple forms of assessment (e.g., clicker, pre/post, and exam questions), and help students make connections with relevant examples. Here we describe an active-learning activity that is suitable for introductory biology classes, addresses several known conceptual difficulties, helps students learn about rising global atmospheric carbon dioxide levels, and connects learning to local photosynthetic organisms that are familiar to students and economically important to their community. Previous work has shown that focusing on locally important organisms and issues allows students to connect their learning to real world problems, helping them move towards understanding biology as a system of interrelated ideas, instead of unrelated concepts (17). In addition, when students are given open-ended questions about drawing ecosystems, they will often draw environments that are more familiar to them, suggesting that these familiar systems may help to scaffold new knowledge (11). Information about timber, potatoes, and sugar kelp in Maine is included in the lesson, but we encourage users of this activity to adapt the examples to align with the needs of their students and we have included resources with relevant information for other regions.
Intended Audience
This lesson is intended for undergraduate introductory biology courses for majors and non-majors. It was taught to students in 11 different biology courses (ranging from 12 to 192 students) at six campuses throughout the University of Maine System (campus locations: Farmington, Fort Kent, Machias, Orono, Presque Isle, and Portland).
Required Learning Time
This lesson is designed for a 75-minute class period, although faculty taught it in different length classes with an average time of 70 minutes.
Pre-requisite Student Knowledge
Delivery of this lesson is not contingent upon a particular set of background knowledge and can be adapted to many contexts. However, some of our instructors found it helpful for students to have been exposed to the conservation and transformation of matter and energy, making and interpreting graphs, unit conversions, and the basic components of the photosynthetic equation.
Pre-requisite Teacher Knowledge
We recommend that instructors know the processes of photosynthesis and transformation of energy and matter in biological systems at a level typically presented in introductory biology textbooks. Instructors can choose to use the lesson as is, and discuss economic issues in Maine, or adjust the examples to be representative of economic systems in their area. For example, timber harvesting occurs in many locations, kelp farming is common in both the Atlantic and Pacific Oceans, and potato and other similar crops are grown in multiple regions.
We also suggest that faculty learn about the Mauna Loa Observatory (https://www.esrl.noaa.gov/gmd/ccgg/trends/full.html) and explore the Environmental Change Model within the Climate Reanalyzer Tool (http://cci-reanalyzer.org/), which will allow instructors to focus on different regions of the world and model the impacts increased global temperature may have on various biomes. Faculty can search local newspapers and brochures from research facilities for information on the impact of rising carbon dioxide levels on their communities. Examples from Maine include: http://bangordailynews.com/2017/02/06/news/state/maines-coastal-waters-are-unhealthy-from-carbon-acidity-are-seaweed-gardens-the-answer/, https://www.bigelow.org/science/topics/ocean-acidification.html, http://www.islandinstitute.org/program/marine-programs/climate-change. Finally, it is helpful for instructors to walk through the entire presentation, particularly practicing the final calculation for how many pine trees it takes to sequester the carbon dioxide produced by the average household in one year (http://www.botany.org/PlantTalkingPoints/CO2andTrees.php#check).
We also advise that instructors identify common conceptual difficulties among their students by using a tool such as the EcoEvo-MAPS assessment (7). EcoEvo-MAPS targets foundational concepts in ecology and evolution, and asks students to evaluate a series of predictions, conclusions, or interpretations as likely or unlikely to be true given a specific scenario. We administered the EcoEvo-MAPS to 715 students from six universities throughout the University of Maine System (Farmington, Fort Kent, Machias, Orono, Presque Isle, and the University of Southern Maine in Portland). Incorrect thinking across the UMaine system included 68% of the students stating that plants take up carbon from the soil, 72% stating that carbon dioxide is used as the source of energy in photosynthetic organisms, and 63% thinking that elements (such as phosphorus, potassium, nitrogen) are direct sources of energy.
SCIENTIFIC TEACHING THEMES
Active Learning
In this lesson students participate in several active learning activities including talking in discussion groups, answering and asking questions, discussing clicker questions, and filling out a worksheet (Supporting File S1: Presentation Slides With Instructor Notes; Supporting File S2: Student Worksheet). To document the types of instructional practices used, we analyzed instruction in nine of the 11 classrooms using the Classroom Observation Protocol for Undergraduate STEM (COPUS) (Figure 1) (18).
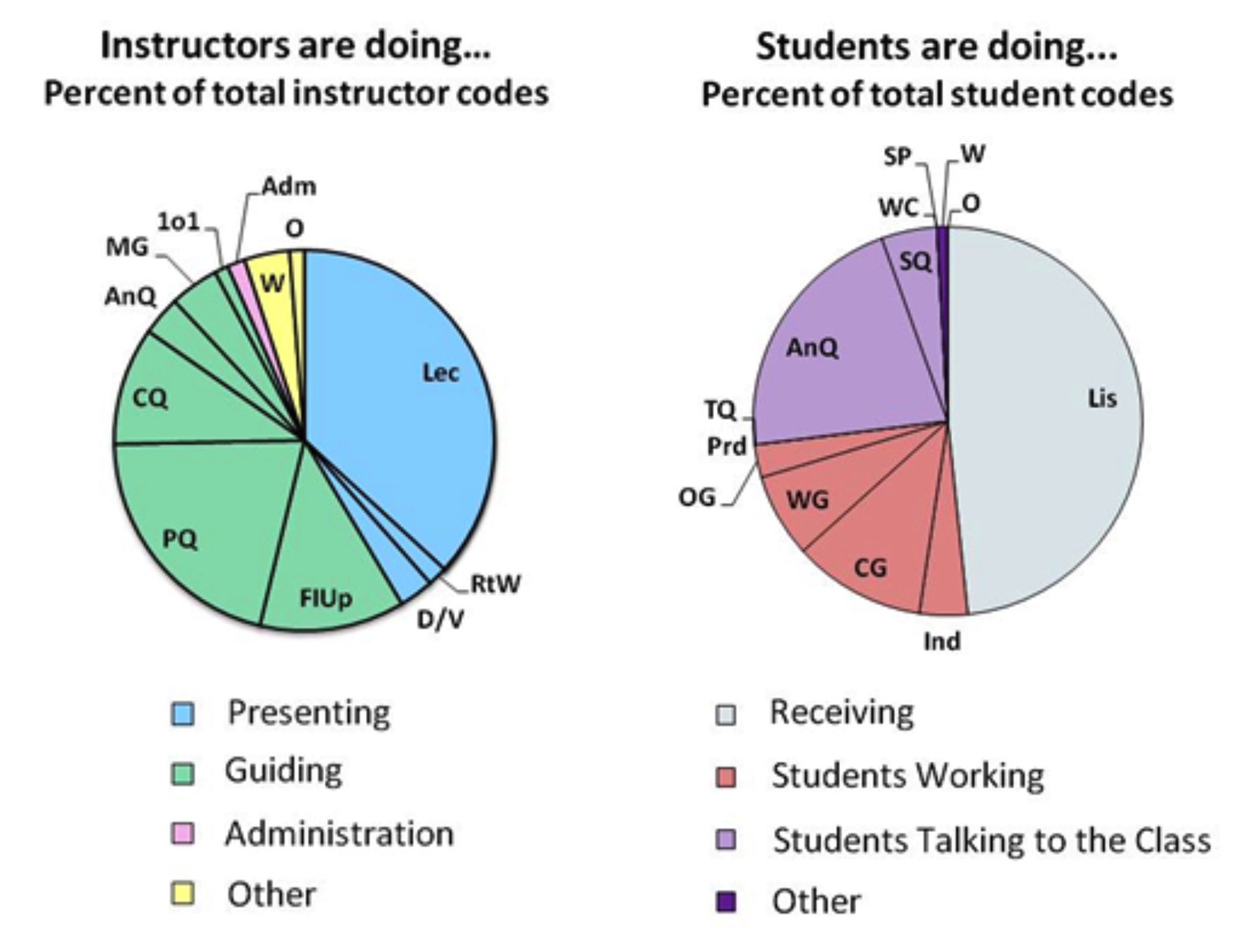
Figure 1. Average collapsed Classroom Observation for Undergraduate STEM (COPUS) (18, 19) codes from 11 classes throughout the University of Maine System. Collapsed instructor codes include: Presenting (Lec-Lecturing, RtW-Real Time Writing, D/V- Demonstration/Video), Guiding (FlUp- Following-up, PQ-Posing Question, CQ-Clicker Question, AnQ-Answering Question, MG-Moving and Guiding, 1o1-One-on-one Instruction), Administration (Adm-Administration), and Other (W-Waiting, O-Other). Collapsed student codes include: Receiving (Lis-Listening), Students Working (Ind-Individual Work, CG-Clicker Question Discussion, WG-Worksheet Group Work, OG-Other Group Work), Students Talking to Class (AnQ-Answering Questions, SQ- Student Question, WC-Whole Class Discussion), and Other (Waiting, Other)
Observers using COPUS mark specific instructor and student behaviors as they occur (such as lecturing and listening, respectively). The COPUS codes can be grouped into broad categories for instructors (i.e., Presenting, Guiding, Administration, and Other) and for students (i.e., Receiving, Students Talking to Class, Students Working, and Other) (19). During this lesson, more than 50% of the instructor codes were "Guiding," which include activities such as posing questions, following up with students, moving and guiding around the classroom, and answering student questions. For the students, approximately 25% of the codes were "Talking to the Class," which includes activities such as asking and answering questions and making predictions. Another 25% of student codes were "Students Working" which describes time spent answering clicker questions, and working individually or with a group.
Assessment
Students were assessed with formative and summative questions aligned to the learning objectives. Formative questions included think-pair-share and in-class multiple-choice questions. Although instructors used different methods to poll student answers to multiple-choice questions (including colored cards, hand raising, and clickers) all of these types of questions have been labeled "Clicker Questions" throughout the lesson. Supporting File S3: Clicker Questions lists the multiple-choice questions and the student response data from one class that used clickers. Typical student answers expressed during the think-pair-share portions of the activity are included in the Progressing Through the Lesson section.
Summative assessment of student thinking was measured using multiple-choice and constructed-response pre/post-test questions, as well as exam questions. The pre/post-test was administered online and contained five multiple-choice questions and two constructed response questions (Supporting File S4: Pre/Post Test Questions). Students were asked to complete the assessment questions to the best of their ability without the help of outside sources.
The two constructed response questions on the pre/post-test were developed for previous assessments to elicit common student conceptual difficulties by asking students to explain the gain in biomass as a seed grows into a maple tree and how planting small quantities of corn seeds can yield exponentially more corn by the end of a single season (20,21). The student answers to the constructed response questions were scored using computer-based lexical analysis tools developed by the Automated Analysis of Constructed Response (AACR) group (21, 22, http://create4stem.msu.edu/project/aacr), which categorizes student answers about the source of biomass. The model detects student writing about carbon dioxide, water, and nutrients, and combinations of these choices. Two authors (MKS and EST) independently coded student answers that did not include these sources of biomass into three additional categories: a different incorrect source of biomass (e.g., sunlight, cell division, soil), nothing about biomass, or an unclear source of carbon (e.g., carbon in the atmosphere and in the soil). The authors discussed any discrepancies and agreed on the final coding.
Students were asked to complete the pre-test in the three days preceding the lesson. Students were given approximately five days to complete the post-test, starting two days after the lesson. Finally, six of the 11 classes included three common questions on their final exam (Supporting File S5: Exam Questions).
Inclusive Teaching
This activity includes a variety of active learning instructional techniques (e.g., think-pair-share, clicker questions) because the active engagement of college students in STEM classes has been shown to increase student performance and decrease the failure rate when compared to classes where the predominant instructional mode is traditional lecturing (23). Additional work has revealed that active learning also decreases the achievement gap for first-generation and other underrepresented students (24). Overall, 41.6% of the full time students in the University of Maine System are first-generation students (https://staticweb.maine.edu/wp-content/uploads/2016/02/First-Generation-Report-to-the-Legislature-2015.pdf?565a1d); therefore, it was important to our group that we use instructional techniques that support their learning.
We also used examples that are economically relevant to the state of Maine throughout the lesson, and observed that students became more engaged when the discussion included an organism important to their community. For example, when students with experience in the timber industry were shown predicted changes in tree species community structure that correlated with an increase in global temperature, they commented on the potential economic disadvantages to their area. Students living in the potato farming region of the state commented on the specifics of how fertilizers are used and the effects these chemicals have on their community over time. Finally, at a campus near the coast, students connected the lesson to research on sugar kelp that was being conducted in their department.
Although the examples in this lesson focused on Maine industries, we encourage instructors to modify the lesson to connect it to economically relevant photosynthetic organisms in their own area. We used organisms that are grown in a variety of ways with both terrestrial (potato and timber) and aquatic (sugar kelp) examples to provide a variety of contexts in which students could apply photosynthetic concepts. To extend this work into other regions, instructors can consult data about local crop production (e.g. https://www.nass.usda.gov/Charts_and_Maps/; https://ipad.fas.usda.gov/), guides focused on aquatic photosynthetic organisms (e.g. https://aquaplant.tamu.edu/plant-identification/; http://www.algaebase.org/), and information about farming in the ocean (e.g. https://ideas.ted.com/vertical-ocean-farms-that-can-feed-us-and-help-our-seas/; https://www.npr.org/sections/thesalt/2017/08/22/542903378/scientists-hope-to-farm-the-biofuel-of-the-future-in-the-pacific-ocean; https://www.frontiersin.org/articles/10.3389/fmars.2017.00100/full).
LESSON PLAN
This lesson is designed for a 75-minute class period (see Table 1 for the progression through the lesson with approximate timing). The intention of this lesson is to address common conceptual difficulties regarding the role of light, carbon dioxide, and nutrients in photosynthetic organisms; expose students to the potential consequences of increasing global carbon dioxide levels in the atmosphere; and connect what they are learning in class to local issues that impact their communities.

Table 1. Energy and Matter - Teaching Timeline. Progression through the lesson with approximate timestamps. Classroom-discussion open-response opportunities are labeled “TPSQ” for think-pair-share questions and clicker question opportunities are labeled “CQ.”
Progressing through the lesson
1. Introduction and assessing prior knowledge
The lesson begins with the instructor orienting student thinking towards photosynthetic organisms and reminding students that energy and matter play key roles in ecosystems. Because the lesson focuses on photosynthetic organisms, students are given the opportunity to recall some of what they already know about these processes.
TPSQ1: What factors are needed for plants to live and grow?
Students are encouraged to brainstorm answers to the first question on their worksheet with their peers (Supporting File S1: Presentation Slides with Instructor Notes, Slide 1; Supporting File S2: Student Worksheet, Question 1). Once students have come up with a short list of answers, the instructor asks for examples from groups to share with the class. In our classrooms answers included light, nutrients, carbon dioxide, and water. The instructor explains that these factors are important for understanding the role of matter and energy, which leads into the rest of the lesson.
2. Learning outcomes and economically important systems
The instructor begins by explaining the learning goals and objectives for the lesson (Supporting File S1: Presentation Slides with Instructor Notes, Slide 2). The instructor explains that the lesson will be taught in the context of three economically important photosynthetic organisms in Maine: timber, potatoes, and sugar kelp (Supporting File S1: Presentation Slides with Instructor Notes, Slide 3). These examples can be exchanged for economic systems of other areas; however, we suggest using both terrestrial and aquatic organisms to allow students to practice transferring their knowledge to novel situations. This introduction also provides an opportunity for instructors to ask students what they already know about the economically relevant systems in order to gauge the experience and interest level of the class. To transition to the next section of the lesson, the instructor can begin by sharing additional information about the timber industry and how it contributes to the economy.
3. Where do trees get their biomass?
This section of the lesson focuses on where plants acquire their biomass, using the timber industry as the economically relevant example.
CQ1: Each year, Maine's forest industry harvests 6-7 million cords of wood to build homes, make furniture, paper, and other products. Which of the following processes contributes the most to the increase in timber biomass?
This question is based on a previously developed assessment question to address student conceptual difficulties about the increase in biomass of photosynthetic organisms (20). Using clickers, or a similar system, students choose from the following answers: A) absorption of mineral substances from the soil via the roots; B) absorption of organic substances from the soil via the roots; C) incorporation of CO2 gas from the atmosphere into molecules by leaves; D) incorporation of H2O from the soil into molecules by green leaves; or E) absorption of solar radiation into the leaf (Supporting File S1: Presentation Slides with Instructor Notes, Slide 4). This question asks about a known conceptual difficulty, and was answered correctly (answer C) by only 25% of the students in the class that used clickers (Supporting File S3: Clicker Questions). Before discussing further, the instructor can comment on the spread of student answers to the clicker question, and show a video in which members of the public are asked a similar question and incorrectly answer that sunlight provides biomass (Veritasium "Where Do Trees Get Their Mass From" https://www.youtube.com/watch?v=2KZb2_vcNTg) (Supporting File S1: Presentation Slides with Instructor Notes, Slide 5). The video also discusses the historical J.B. van Helmont willow tree experiment, which provided evidence that plants do not get their biomass from carbon in the soil (14,15), and explains that the biomass is largely accumulated from carbon dioxide in the air. After watching the video, students are given the clicker question (CQ1) a second time, and encouraged to discuss in groups before voting again (Supporting File S1: Presentation Slides with Instructor Notes, Slide 7). In the class that used clickers, the majority of students (92%) now selected the correct answer C) (Supporting File S1: Presentation Slides with Instructor Notes, Slide 6; Supporting File S3: Clicker Questions).
TPSQ2: When asked "Where do trees get the mass to grow big?" many in the video answered, "the sun." Talk to your neighbor about why this is incorrect.
Students are given 2-4 minutes to discuss this question in their groups, followed by time to share ideas with the class (Supporting File S1: Presentation Slides with Instructor Notes, Slide 8). In our classes, students reasoned that trees could not get their mass from the sun because: "if this were true, trees would shrink in the winter" and "light does not have mass." The instructor follows up with information about the first law of thermodynamics, explaining that energy from the sun stays as energy throughout photosynthesis, it is just converted into chemical energy. Similarly, matter is converted from carbon dioxide into biomass (often in the form of glucose, cellulose, and starch), but it cannot be changed into energy (Supporting File S1: Presentation Slides with Instructor Notes, Slides 9-10).
4. Identifying and discussing seasonal patterns of atmospheric carbon dioxide and the relationship with photosynthesis
This section of the lesson focuses on how atmospheric carbon dioxide levels affect the process of photosynthesis. The instructor begins by introducing the Mauna Loa Observatory in Hawaii and discussing the data collection of atmospheric carbon dioxide levels over many years (Supporting File S1: Presentation Slides with Instructor Notes, Slide 11). If time allows, some instructors may ask students why they think this location may be ideal for atmospheric carbon dioxide monitoring, and discuss the work of C. David Keeling (https://www.esrl.noaa.gov/gmd/co2conference/Reporters/Nature50yearsonTheKeelingCurvele.pdf, https://www.esrl.noaa.gov/gmd/ccgg/info.html, https://www.esrl.noaa.gov/). Students are then asked to plot 2012 Mauna Loa atmospheric carbon dioxide data on their worksheet (Supporting File S1: Presentation Slides with Instructor Notes, Slide 12; Supporting File S2: Student Worksheet, Question 2). Once students have completed their individual plots, they can compare their drawings with peers and the example plot presented (Supporting File S1: Presentation Slides with Instructor Notes, Slide 13).
TPSQ3: Hypothesize why atmospheric CO2 levels are lowest in September and highest in May.
Students are given time to discuss their hypotheses about why atmospheric carbon dioxide levels are lowest at the end of the summer and highest in the beginning of spring (Supporting File S1: Presentation Slides with Instructor Notes, Slide 13; Supporting File S2: Student Worksheet, Question 3). After discussion, the instructor asks students to share their thoughts with the class. In our classes these ideas included "plants use more carbon dioxide when their leaves are out" and "there is more sunlight in the summer months, so the plants are absorbing more carbon dioxide." The instructor can then follow up by explaining that in the Northern Hemisphere photosynthetic organisms, such as trees, are more active in the summer, which results in the removal of more carbon dioxide from the atmosphere. Conversely, these organisms are less active in the winter, which allows for carbon dioxide levels to build up in the atmosphere (Supporting File S1: Presentation Slides with Instructor Notes, Slide 14). If time allows, instructors can then ask students to predict how this plot would look different in the Southern Hemisphere, and explain that seasonal variations in atmospheric carbon dioxide are more pronounced in the Northern Hemisphere because of the larger landmass, and therefore greater plant biomass (Supporting File S1: Presentation Slides with Instructor Notes, Slide 15).
The instructor then shows plots of atmospheric carbon dioxide over longer time scales and asks students to predict how these increases may affect plants and photosynthesis (Supporting File S1: Presentation Slides with Instructor Notes, Slides 16-17). In our classes, students often said that plants could use the increased carbon dioxide to perform more photosynthesis, but hypothesized that there would not be enough plants to offset the excess carbon dioxide. The instructor can then share misleading popular press articles that state that rising global carbon dioxide levels will be good for plants and therefore benefit our world. A quick search on the internet (ex. "rising CO2 is good for plants" "CO2 myths") will uncover several articles that emphasize this conclusion. We ask students to consider titles of these articles and identify some things that the articles could be omitting (Supporting File S1: Presentation Slides with Instructor Notes, Slide 18, Supporting File S2: Student Worksheet, Question 4). Students in our classes said that these articles were most likely overlooking information about the correlation between increased atmospheric carbon dioxide and changes in precipitation patterns, pest ranges, and temperature. After discussing these potential effects on a global scale (Supporting File S1: Presentation Slides with Instructor Notes, Slide 19), the instructor relates this discussion back to the timber economy. Students observe a map of the biome composition throughout New England and are asked to make a prediction about how the biome distribution will change over time as temperature changes (Supporting File S1: Presentation Slides with Instructor Notes, Slide 20). Using the Climate Reanalyzer Tool, (http://cci-reanalyzer.org/) students are shown a model of changes in forest composition over time as the temperature increases two degrees Celsius and can see that some tree species are predicted to disappear and other species of trees will move north. Although we showed students a model of changes in New England, instructors can select other regions that are more locally relevant. After showing the model, some instructors, especially in classes where many students are particularly connected with the timber industry, chose to spend more time discussing specific types of trees that would be affected, and how that would affect both the biome and the industry.
5. Role of nutrients
Next, students are asked to think back to their initial list of requirements for plant growth (TPSQ1) and identify which factors the class has yet to discuss (i.e., nutrients) (Supporting File S1: Presentation Slides with Instructor Notes, Slide 21). In our lesson, students learned about the role of nutrients in the context of potato farming (Supporting File S1: Presentation Slides with Instructor Notes, Slide 22). Here, the instructor can share background knowledge and additional facts concerning the industry chosen for this section of the lesson. Instructors may also ask students to offer examples of the nutrients plants need to grow (i.e., nitrogen, phosphorus, potassium) and where we often see this combination of elements (i.e., fertilizer).
CQ2: A potato farmer adds nutrients in the form of fertilizer to the soil around her plants. What does the fertilizer provide for the plants?
Students are asked to individually answer a clicker question about what fertilizers provide for plants. Answer choices include: A) energy to the plant, B) chemicals for plant cells to function, C) carbon to help the plants grow, or D) all of the above. In the class that used clickers, only 24% of the students correctly answered B, while 69% of the students incorrectly answered D (Supporting File S1: Presentation Slides with Instructor Notes, Slide 23; Supporting File S3: Clicker Questions). Students then discussed their answers with peers and voted again. This time, 47% of the students correctly answered B but 51% still incorrectly answered D (Supporting File S3: Clicker Questions). The instructor can then ask students about how they arrived at their answers (Supporting File S1: Presentation Slides with Instructor Notes, Slide 24).
The instructor explains that when 3000 students from 34 institutions were asked a similar question during a previous study, 57% thought that fertilizers were a direct source of energy for plant growth (7). Students are asked, "Why do so many students think this?" and are then shown an image of a fertilizer bag, with advertisements that imply that it adds energy to plants (Supporting File S1: Presentation Slides with Instructor Notes, Slide 25). The instructor can discuss how including terms such as "energy buttons" on packaging can be misleading, and contribute to the conceptual difficulty. The instructor then discusses the differences between macro- and micronutrients (Supporting File S1: Presentation Slides with Instructor Notes, Slide 26) and explains that plants need nutrients for the building blocks of amino acids, ATP, and other molecules in the cell (Supporting File S1: Presentation Slides with Instructor Notes, Slide 27).
CQ3: Over time, what happens to the majority of nutrients added to a Maine potato farm?
Students are given information about the total nutrients added to Maine farms each year (document found on the maine.gov website by searching "fertilizer tonnage report") (Supporting File S1: Presentation Slides with Instructor Notes, Slide 28). Next, they individually answer a clicker question about what happens to the majority of nutrients added to a Maine potato farm. Choices include: A) the nutrients accumulate in the soil, B) the nutrients provide energy for next year's growth, C) the nutrients are removed from the field, or D) the nutrients are locally recycled in the ecosystem (Supporting File S1: Presentation Slides with Instructor Notes, Slide 29). Even after discussing the question with peers, only 25% of the students in the class that used clickers correctly answered C, the majority of the nutrients are removed from the field (Supporting File S3: Clicker Questions). Instead, most students incorrectly answered D, indicating that they think most nutrients remain where they are placed (Supporting File S3: Clicker Questions). The instructor can then ask students to talk about their answer choices and explain that in agricultural systems, such as a potato farm, the majority of the nutrients are removed from the field either through harvesting or runoff to other areas (Supporting file S1: Presentation Slides with Instructor Notes, Slide 30).
TPSQ4: If nutrients are important, why is it often not necessary to add nutrients (through fertilizer) to the forests?
We also asked students about what happens to nutrients in forests (Supporting File S1: Presentation Slides with Instructor Notes, Slide 31). Students discuss the question with peers and shared answers with the class. Many students in our classes brought up detritus and discussed how, unlike a field, forests accumulate natural compost over time. Students thought that the large number of trees and other plants in a forest could perhaps decrease the amount of nutrient runoff. The instructor can then follow up with an explanation about the decomposition of organic materials in a forest.
6. Application to aquaculture
The next section of the class allows students to apply what they have learned about terrestrial environments to an aquatic environment. For our lesson, we selected sugar kelp, which is farmed off the coast of Maine (Supporting File S1: Presentation Slides with Instructor Notes, Slide 32). Students are often unfamiliar with uses for processed kelp, so we provided information about the incorporation of kelp in beauty products, food, and vitamin supplements (Supporting File S1: Presentation Slides with Instructor Notes, Slide 33). Instructors can give students information about the structure of kelp (Supporting File S1: Presentation Slides with Instructor Notes, Slides 34 and 35) and compare kelp to a potato plant to emphasize that, even though kelp are photosynthetic algae rather than plants, they are similar in that they are both photosynthetic organisms.
CQ4: The pictures below are of sugar kelp grown in an aquaculture farm in Walpole, Maine. Picture A was taken at the start of the growing season, and picture B is the same line of sugar kelp three months later. Which of the following is the most likely source for the increase in sugar kelp biomass over this time period?
Students are then shown two photos of sugar kelp, one at the beginning of the growing season and the other at the end, and asked which of the following is responsible for the increase in biomass: A) nutrients, B) water, C) light, or D) CO2 (Supporting File S1: Presentation Slides with Instructor Notes, Slide 36). Clicker Questions 1 and 4 (CQ1 and CQ4) address the same concept, but CQ4 changes the system to an aquatic environment where carbon dioxide is dissolved in water. After discussing this question with peers, 73% of the students in the class that used clickers correctly answered D (Supporting File S1: Presentation Slides with Instructor Notes, Slide 37; Supporting File S3: Clicker Questions). The instructor can reinforce that carbon dioxide is the main source of biomass for all photosynthetic organisms, and the process of photosynthesis is similar in sugar kelp and the terrestrial plants described earlier in the lesson (Supporting File S1: Presentation Slides with Instructor Notes, Slides 38 and 39). The instructor can then show that dissolved carbon dioxide in seawater is rising as atmospheric carbon dioxide is rising, and that consequently, the pH of the oceans is decreasing (Supporting File S1: Presentation Slides with Instructor Notes, Slides 40 and 41). The instructor then shares information about how kelp farms can mediate ocean acidification by absorbing dissolved carbon dioxide and a news story about the importance of seaweed gardens (Supporting File S1: Presentation Slides with Instructor Notes, Slides 42 and 43) (http://bangordailynews.com/2017/02/06/news/state/maines-coastal-waters-are-unhealthy-from-carbon-acidity-are-seaweed-gardens-the-answer/).
7. Synthesis
The lesson concludes with information about how photosynthetic plants relate to fossil fuels (Supporting File S1: Presentation Slides with Instructor Notes, Slide 44). The instructor explains that fossil fuels are relics of ancient plant biomass and are rich in carbon. Plants absorb carbon dioxide, which is converted to biomass, so the burning of fossil fuels releases this carbon back into the atmosphere (Supporting File S1: Presentation Slides with Instructor Notes, Slide 44).
TPSQ5: How many pine trees does it take to sequester the CO2 produced by your household in a year?
To help students estimate how much carbon dioxide their household produces in a year, the instructor can use an activity modified from the Botanical Society of America (http://www.botany.org/PlantTalkingPoints/CO2andTrees.php#check). In this activity, students are given the necessary variables to calculate how many trees are needed to offset the carbon footprint of an average household's energy usage in one year (Supporting File S1: Presentation Slides with Instructor Notes, Slide 45; Supporting File S2: Student Worksheet, Question 5). Students determine that it takes 245 trees to sequester the carbon associated with an average home, which typically sparks additional conversation as they begin to think about their energy usage in terms of numbers of trees (Supporting File S1: Presentation Slides with Instructor Notes, Slide 46).
TEACHING DISCUSSION
A variety of methods were used to assess the effectiveness of this lesson, including formative clicker and think-pair-share questions during the lesson, as well as summative pre/post-test and exam questions (Table 2). Here we discuss the summative questions; student answers to the formative questions are presented in the Progressing Through the Lesson section.
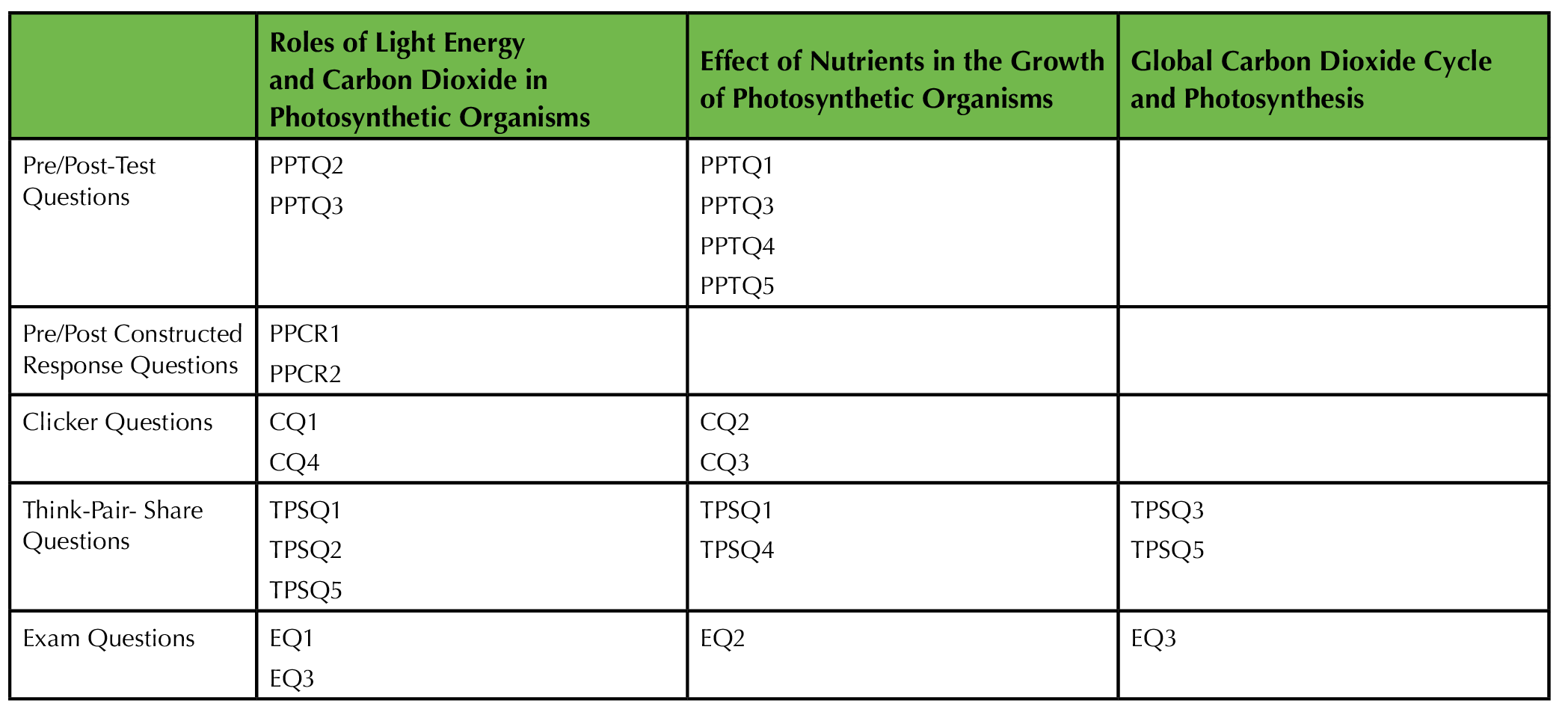
Table 2. Energy and Matter - Assessments used to examine student understanding of energy and matter in photosynthesis arranged by learning objective. Question types are abbreviated PPTQ (Pre/Post-Test Question), PPCR (Pre/Post Constructed Response), CQ (Clicker Question), TPSQ (Think-Pair-Share Question), and EQ (Exam Question).
Student Performance and Conceptual Difficulties
Pre/Post Multiple Choice Questions
Students in all 11 courses completed both pre- and post-test questions (abbreviated PPTQ for Pre/Post-Test Questions) (Supporting File S4: Pre/Post-Test Questions). The questions address two of the learning objectives: 1. Describe the roles of light energy and carbon dioxide in photosynthetic organisms, and 2. Identify the effect of nutrients on the growth of photosynthetic organisms. Here we report the results for students who completed both the pre- and post-test and agreed to the informed consent (n=92). Across all 11 classes, the overall average pre-test score was 25% and the average post-test score was 65%. To calculate the normalized gain for overall scores on the pre/post-test multiple choice questions, we used the following formula (25):

equation
The normalized gain for the pre/post-test is
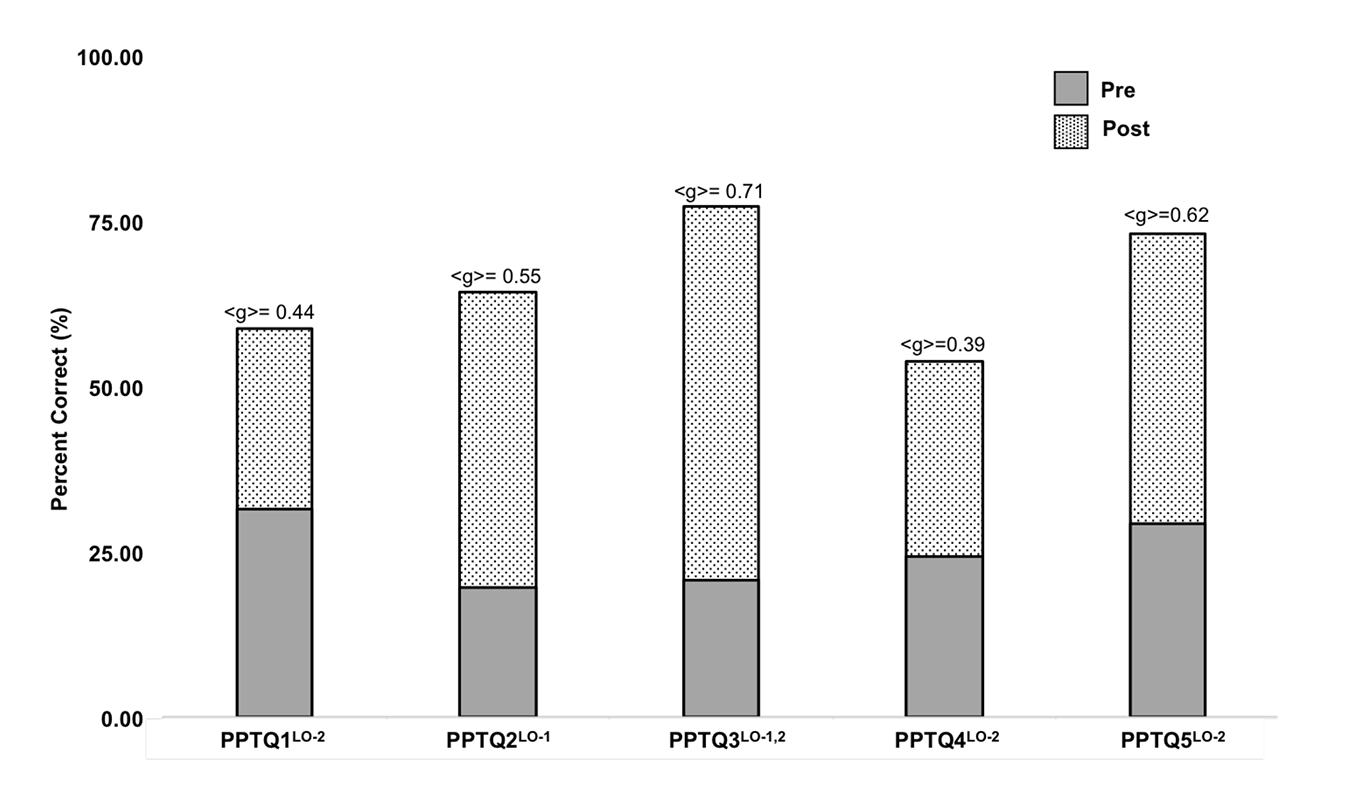
Figure 2. Student percent correct before and after instruction on pre/post-test questions (PPTQ). Normalized gain <g> is provided for each question. Questions PPTQ1-3 were answered by 92 students, PPTQ 4-5 were added later and answered by 41 students. Targeted learning objectives are indicated for each question as: LO-1 Describe the roles of light energy and carbon dioxide in photosynthetic organisms and/or LO-2 Identify the effect of nutrients on growth of photosynthetic organisms.
The pre/post-test questions focus on different student conceptual difficulties and learning objectives. Two questions (PPTQ1 and PPTQ4) are true/false questions, which state that photosynthetic organisms use nutrients as energy (Supporting File S4: Pre/Post-Test Questions). The first question (PPTQ1) uses seaweed as an example of a marine photosynthetic organism and by the end of this lesson 58% of the students in our classes correctly answered that this statement is false (Figure 2). The later question (PPTQ4) asks about the terrestrial crop blueberries and by the end of the lesson, 54% of the students in our classes correctly answered this question as false (Figure 2). Although there were positive learning gains for both questions from pre- to post-test, a subset of students still struggled with whether or not nutrients provide energy to photosynthetic organisms.
PPTQ2 and PPTQ3 both ask students about the source of biomass for photosynthetic organisms (Supporting File S4: Pre/Post-Test Questions). PPTQ2 states that photosynthetic organisms, such as trees, convert sunlight to biomass, which was correctly identified as false by 64% of students in our classes on the post-test (Figure 2). PPTQ3 includes two pictures, one showing plants growing hydroponically and one showing plants growing in the soil. Students are asked to determine where each of the plants gets their biomass. On the post-test, 77% of the students in our classes correctly answered that the plants in both pictures get biomass from carbon dioxide in the atmosphere (Figure 2). These questions show some of the highest post-test percent correct percentages and learning gains.
Finally, PPTQ5 asks students to predict what happens to the majority of nutrients added to a fertilized blueberry field (Supporting File S4: Pre/Post-Test Questions). On the post-test in our classes, 73% of the students correctly answered that the majority of the nutrients are removed from the system (Figure 2).
Pre/Post Constructed Response Questions
The pre/post-test also included two constructed response questions aligned with the first learning objective; describe the roles of light energy and carbon dioxide in photosynthetic organisms.
(Supporting File S4: Pre/Post-Test Questions). Pre/Post Constructed Response 1 (PPCR1) asks students to explain the gain in biomass as a seed grows into a maple tree and PPCR2 asks students how planting small quantities of corn seeds can yield exponentially more corn by the end of a single season. These questions were developed from earlier studies on student thinking about energy and matter in photosynthesis (20,21). Student responses to these questions were analyzed with a model created by the Automated Analysis of Constructed Response (AACR) project (http://create4stem.msu.edu/project/aacr/questions). The AACR model uses word choice analysis to determine whether students talk about the correct (i.e., carbon dioxide) or incorrect (e.g., water, nutrients, etc.) source of biomass in their answers. To develop the automatic scoring rubric for the maple tree and corn plant AACR questions, the computer algorithm was compared to scoring by content experts for 832 student responses. The kappa values comparing the computer algorithm to the experts were as follows: 0.917 for identifying carbon dioxide/carbon as the correct source of biomass, 0.858 for incorrectly identifying water as the source of biomass, and 0.694 for incorrectly identifying nutrients from the soil is (http://create4stem.msu.edu/project/aacr/questions).
The pre-test data show that for both questions, students rarely identify carbon dioxide alone as the correct source of the biomass for a growing plant (Figure 3, A and C). Instead, they often write about some combination of carbon dioxide, water, and nutrients; and include additional incorrect sources of biomass such as sunlight, soil, and cell division. The data show that on the post-test for both PPCR1 and PPCR2, students were more likely to talk about the correct source of biomass (i.e., carbon dioxide) and less likely to include other sources in their answers (Figure 3, B and D).
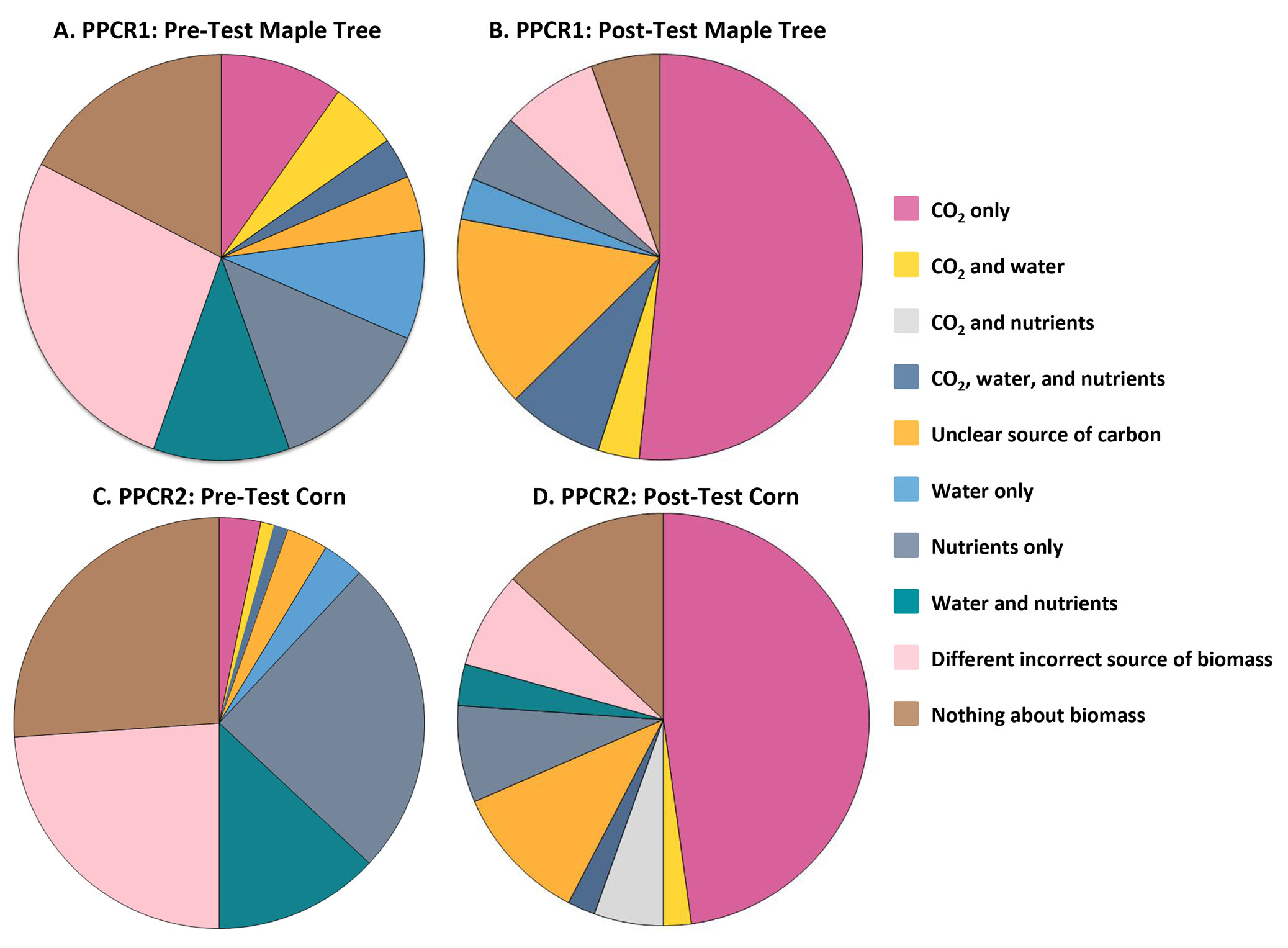
Figure 3. Student answers to two constructed response questions. A) Pre- and B) post-test answers to a question about the source of the increase in biomass as a seed grows into a one ton maple tree. C) Pre- and D) post-test answers to a question about the source of biomass that explains how planting small quantities of corn seeds can yield exponentially more corn by the end of a single season.
Exam Questions
Students in six of the 11 classes answered three multiple-choice questions (abbreviated EQ) on their final exam (Supporting File S5: Exam Questions). Here we report the results for students who completed the exam questions and agreed to the informed consent (n=56 for EQ1 and EQ2, and n=65 for EQ3). The first exam question (EQ1) asks students what contributes the most to an increase in potato plant biomass and 66% of the students correctly answered "incorporation of CO2 gas from the atmosphere" (Figure 4). The second exam question (EQ2) asks students to pick out the most accurate name and description for a fictitious fertilizer: A) Energy Buttons: providing the food your plant needs to grow, B) All-Purpose Nutrients: promotes growth in your plants, C) Carbon Capsules: adding carbon to your soil will help the plants grow, or D) Liquid Enhancer: giving your plants the moisture they need to grow. The majority of the students (63%) correctly answered B, while the most common incorrect answer was C (21%) (Figure 4). Finally, for EQ3, students were given a Keeling curve and the lowest point for the 2014 data was marked with a star. Students were asked to predict the season that corresponded to the star, and provide an explanation. Our results showed that students were split between the incorrect answer, B) end of winter, because photosynthetic organisms use less CO2 in the winter (40% of students), and the correct answer, C) end of summer, because photosynthetic organisms use CO2 at higher rates in the summer (48% of students) (Figure 4). Both groups of students selected the correct explanation to go with the corresponding season, but those selecting B incorrectly interpreted the x-axis of the Keeling curve which is time marked by year.
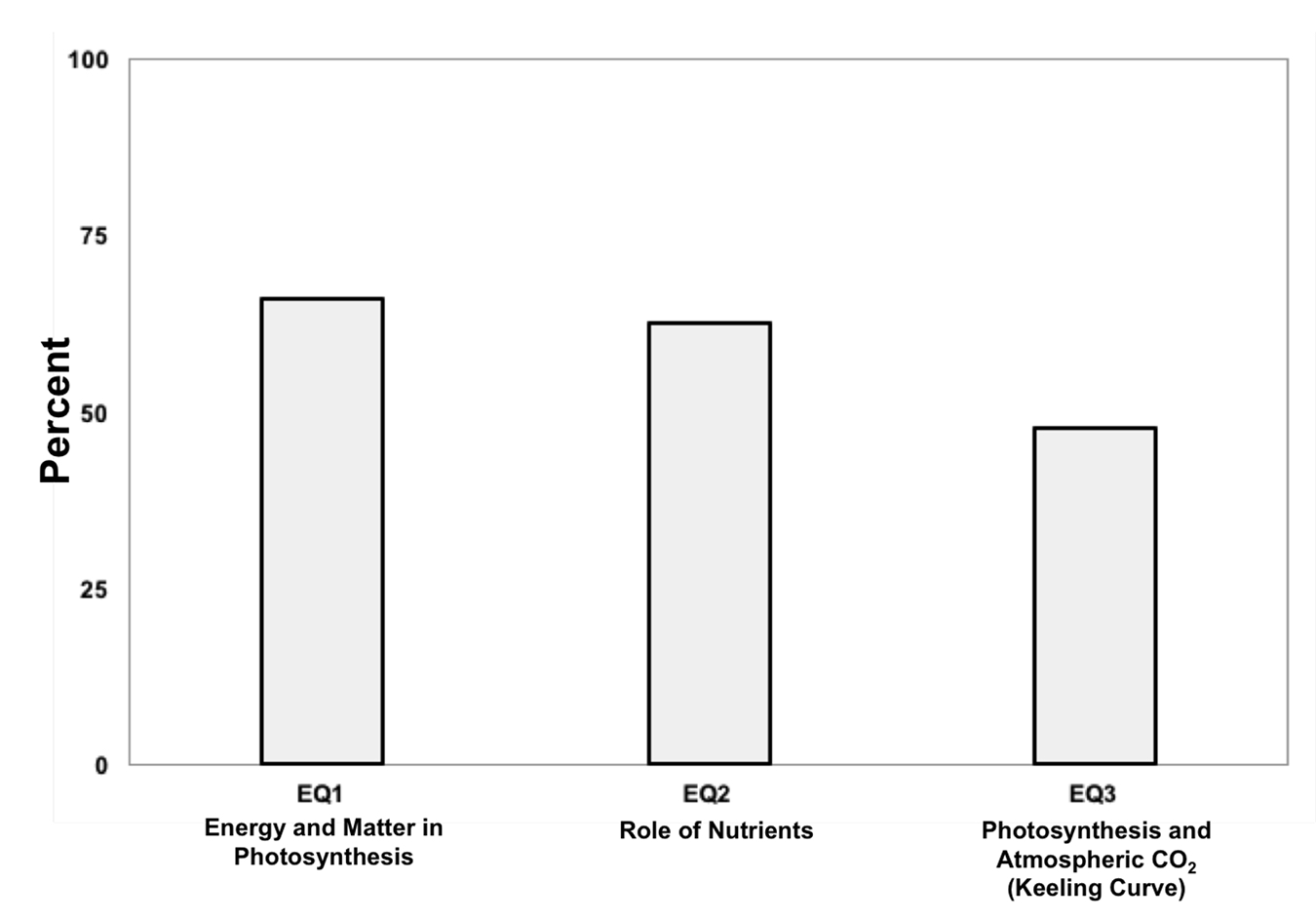
Figure 4. Student percent correct for exam questions (abbreviated EQ).
Additional Suggestions to Enhance Student Learning While Using this Lesson
Based on student answers to formative and summative questions, we make the following recommendations to further improve student learning:
1. Revisit these concepts multiple times.
Understanding the roles light energy, carbon dioxide, and nutrients have in photosynthetic organisms are examples of persistent conceptual difficulties for undergraduate students and confusion about these concepts often continues into their senior year (4,5,6,7,8,9). EcoEvo-MAPS assessment data revealed that the majority of the students throughout the University of Maine System incorrectly think that plants take up carbon from the soil, carbon dioxide is used as the source of energy in photosynthetic organisms, and elements (such as phosphorus, potassium, nitrogen) are direct sources of energy (7). The pre-test assessment and individual student responses to clicker questions in our current study supported the results of the EcoEvo-MAPS, revealing students rarely understood the roles of carbon dioxide, sunlight, and nutrients in photosynthesis across all question types (Figure 2: pre-test multiple choice; Figure 3, A and C: pre-test constructed response; Supporting File S3: Clicker Questions: individual clicker question responses before peer/class discussion). Despite clear gains in conceptual understanding based on post-test scores (Figure 2), constructed response answers (Figure 3, C and D), and exam scores (Figure 4), many students retained their initial conceptual difficulties. Persistent conceptual difficulties can be especially challenging for students to overcome and often require instructors to use a variety of teaching strategies to help correct incorrect ideas (26). Therefore, instructors should continue to revisit these concepts within and across multiple courses (e.g. during photosynthesis, matter and energy, and ecosystem units) and use a variety of methods (e.g., clicker questions, small group activities, homework questions) to provide many opportunities for these difficulties to be corrected.
2. Provide more opportunities for students to learn about global carbon dioxide levels.
The alignment between assessment questions and the learning objectives (Table 2) demonstrates that we have fewer questions that address Learning Objective 3: Describe global cycles in atmospheric carbon dioxide levels and how they relate to photosynthetic organisms. In addition, student performance on the exam question aligned to this learning objective (EQ3) was the lowest across all three exam questions (Figure 4). To address student confusion, we suggest instructors spend more time emphasizing connections between photosynthesis and global carbon dioxide levels. This topic can be emphasized through direct instruction (e.g., reinforcing that the Keeling curve graphs atmospheric carbon dioxide levels), adding additional individual and class discussion questions (e.g., clicker, think-pair-share), and asking associated homework questions that increase students' experience working with these concepts (e.g., asking students to plot atmospheric carbon dioxide data and predict seasons).
3. Contrast nutrient cycling in farmed versus natural environments.
Farmed systems experience different inputs (i.e., fertilizers) and outputs (i.e., harvesting) than natural systems, thereby affecting nutrient cycles. Despite addressing both types of systems in our lesson, student comments suggested that there was continued confusion about the role of nutrients in systems that are not being farmed, especially forest systems where trees are often harvested. We recommend that instructors add further discussion about local nutrient recycling in forests and contrast that to farming situations where fertilizers are typically added to the soil and a large percentage of biomass is removed at the end of each growing season.
Conclusions
This lesson engages students in active learning to address common conceptual difficulties regarding the roles light energy, carbon dioxide, and nutrients have in photosynthetic organisms. Locally relevant examples are used to help students connect their learning to global scale and real world problems (17) (e.g., increasing carbon dioxide). Several inclusive teaching practices are used throughout the lesson, including clicker questions, think-pair-share questions, and a worksheet. This activity leads to student learning gains in the shorter term, as is evident through clicker questions (Supporting File S3: Clicker Questions) and pre/post-test data (Figures 2 and 3), and in the longer term, which can be seen in final exam question results (Figure 4). Making these gains and continuing to build on them in subsequent courses is important because Energy and Matter are key concepts in biology (1,2) and a solid understanding of these concepts is important for understanding issues that have an impact on both local and global scales.
SUPPORTING MATERIALS
- S1. Energy and Matter-Presentation Slides with Instructor Notes
- S2. Energy and Matter-Student Worksheet (does not include in-class multiple choice questions, for use in a class that utilizes a student personal response system such as clickers)
- S3. Energy and Matter-Clicker Questions
- S4. Energy and Matter-Pre/Post Test Questions
- S5. Energy and Matter-Exam Questions
- S6: Energy and Matter-Biome Movie (this movie is associated with Slide 20 in Supporting File S1)
ACKNOWLEDGMENTS
We would like to thank Drs. Kevin Haudek, John Merrill, Rosa Moscarella, and Mark Urban-Lurain for their help analyzing the AACR data. We would also like to thank the students for engaging in the activity and answering the assessment questions.
References
- American Association for the Advancement of Science. 2009. Vision and change in undergraduate biology education: a call to action. Washington, DC: American Association for the Advancement of Science.
- NGSS Lead States. 2013. Next Generation Science Standards: For states, by states. National Academies Press, Washington, DC.
- Cordero EC, Todd AM, Abellera D. 2008. Climate change education and the ecological footprint. American Meteorological Society.
- Anderson CW, Sheldon TH, Dubay J. 1990. The effects of instruction on college nonmajors' conceptions of respiration and photosynthesis. Journal of Research in Science Teaching, 27(8): 761-776.
- Hartley LM, Wilke BJ, Schramm JW, D'Avanzo C, Anderson CW. 2011. College Students' Understanding of the Carbon Cycle: Contrasting Principle-based and Informal Reasoning. BioScience, 61(1): 65-75.
- Boyes E, Stanisstreet M. 1991. Misconceptions in First-Year Undergraduate Science Students about Energy Sources for Living Organisms. Journal of Biological Education 25(3):209-213.
- Summers MM, Couch BA, Knight J, Brownell S, Crowe A, Semsar K, Wright CD, Smith, MK. EcoEvo-MAPS: an ecology and evolution assessment tool for introductory and advanced students. CBE Life Sci Educ, in press.
- Wilson CD, Anderson CW, Heidemann M, Merrill JE, Merritt BW, Richmond G, Sibley DF, Parker JM. 2006. Assessing students' ability to trace matter in dynamic systems in cell biology. CBE Life Sci Educ 5(4):323-331. doi: 10.1187/cbe.06-02-0142.
- Ebert-May D, Batzli J, Lim H. 2003. Disciplinary research strategies for assessment of learning. Bioscience 53:1221-1228.
- Darby BJ, Goodwin BJ. 2014. Using Pathway Maps to Link Concepts, Peer Review, Primary Literature Searches and Data Assessment in Large Enrollment Classes: An example from teaching ecosystem ecology. CourseSource. https://doi.org/10.24918/cs.2014.2.
- Sanford RM, Staples JK, Snowman SA. 2017. The draw-an-ecosystem task as an assessment tool in environmental science education. Sci Educ Civ Engagement 9(1):35-40.
- Kose, S. 2008. Diagnosing Student Misconceptions: Using Drawings as a Research Method. World Applied Sciences Journal 3(2):283-293.
- O'Connell D. 2008. An inquiry-based approach to teaching photosynthesis and cellular respiration. Am Biol Teach 70:6.
- Partington JR. 1936. Joan Baptista van Helmont. Annals of Science 1(4): 359-384.
- Wandersee JH. 1986. Can the history of science help science educators anticipate students' misconceptions? Journal of Research in Science Teaching 23(7):581-597.
- Williams MA, Wassenberg D. 2017. Promoting climate change literacy for non-majors: Implementation of an atmospheric carbon dioxide modeling activity as an inquiry-based classroom activity. CourseSource, 4:1-5.
- Chamany K, Allen D, Tanner K. 2008. Making Biology Learning Relevant to Students: Integrating People, History, and Context into College Biology Teaching. CBE Life Sci Educ 7:267-278.
- Smith MK, Jones FH, Gilbert SL, Wieman CE. 2013. The Classroom Observation Protocol for Undergraduate STEM (COPUS): a new instrument to characterize university STEM classroom practices. CBE Life Sci Educ 12:618-627.
- Smith MK, Vinson EL, Smith JA, Lewin JD, Stetzer MR. 2014. A campus-wide study of STEM courses: New Perspectives on Teaching Practices and Perceptions. CBE Life Sci Educ 13:624-635.
- Parker JM, Anderson CW, Heidemann M, Merrill J, Merritt B, Richmond G, Urban-Lurain M. 2012. Exploring undergraduates' understanding of photosynthesis using diagnostic question clusters. CBE Life Sci Educ 11:47-57.
- Weston M, Haudek KC, Prevost L, Urban-Lurain M, Merrill J. 2015. Examining the impact of question surface features on students' answers to constructed-response questions on photosynthesis. CBE Life Sci Educ 14.
- Haudek KC, Kaplan JJ, Knight J, Long T, Merrill J, Munn A, Nehm R, Smith M, Urban-Lurain M. 2011. Harnessing Technology to Improve Formative Assessment of Student Conceptions in STEM: Forging a National Network. CBE Life Sci Educ 10(2):149-155.
- Freeman S, Eddy SL, McDonough M, Smith MK, Okoroafor N, Jordt H, and Wenderoth MP. 2014. Active learning increases student performance in science, engineering, and mathematics. PNAS 111(23):8410-8415.
- Eddy SL, Hogan KA. 2014. Getting under the hood: how and for whom does increasing course structure work? CBE Life Sci Educ 13:453-468.
- Hake R. 1998. Interactive-engagement versus traditional methods: a six-thousand-student survey of mechanics test data for introductory physics courses. Am. J. Phy. 66:64-74.
- National Research Council. 2012. Discipline-Based Education Research: Understanding and Improving Learning in Undergraduate Science and Engineering. Washington, DC: The National Academies Press. https://doi.org/10.17226/13362.
Article Files
Login to access supporting documents
Using Place-Based Economically Relevant Organisms to Improve Student Understanding of the Roles of Carbon Dioxide, Sunlight, and(PDF | 655 KB)
S1. Energy and Matter-Presentation slides.pptx(PPTX | 24 MB)
S2. Energy and Matter-Student worksheet.docx(DOCX | 91 KB)
S3. Energy and Matter-Clicker questions.docx(DOCX | 8 KB)
S4. Energy and Matter-Pre-post test questions.docx(DOCX | 808 KB)
S5. Energy and Matter-Exam questions.docx(DOCX | 84 KB)
S6. Energy and Matter-Biome movie.gif(GIF | 854 KB)
- License terms
Comments
Comments
There are no comments on this resource.