A flexible, multi-week approach to plant biology - How will plants respond to higher levels of CO2?
Published online:
Abstract
To help introductory biology students understand how plants will respond to higher levels of CO2, we have created a multi-week module consisting of a series of four related laboratory lessons. These lessons allow students to make connections among morphological, physiological, and growth responses of Brassica rapa (Fast Plants®) to low (400 ppm) and high (800 ppm) concentrations of atmospheric carbon dioxide (CO2). Elevated CO2 was chosen as an environmentally relevant variable of high interest to our students as they consider the environment of their futures. However, this module is easily adaptable to test other plant species and/or variables (e.g., light, nutrients, soil contaminants, genotypes, biotic factors, etc.), depending on instructor/student interests and available facilities/equipment. Within our modular framework, students analyze stomatal densities, photosynthetic and respiratory rates, foliar protein concentrations, and growth/allocation patterns. Progressive, weekly analysis of B. rapa responses to high and low CO2 concentrations allows students to apply their findings and make predictions about plant responses in subsequent lessons. After all responses are measured, this framework facilitates student synthesis as they describe the interconnectedness of the measured processes and reflect on how these responses contribute to "how plants will respond to higher levels of CO2."
Citation
Muilenburg, V., Supanich, K., Scogin, S., Bultman, T., Li, J., Hertel, L., and Winnett-Murray, K. 2018. A flexible, multi-week approach to plant biology - How will plants respond to higher levels of CO2?. CourseSource. https://doi.org/10.24918/cs.2018.13Society Learning Goals
Plant Biology
- Information Processing/Signal Transduction
- How do plants detect, process, and interpret information from the environment?
Lesson Learning Goals
Students will:- Understand the scientific method, including asking questions, formulating hypotheses, gathering and analyzing data, predicting outcomes, and displaying results.
- Learn how to keep a scientific lab notebook.
- Learn about the components of a scientific paper and develop skills in literature research and scientific writing.
- Develop collaborative skills.
- Understand basic statistical analysis of biological data.
- Gain basic knowledge about plant anatomy/physiology and better understand the relationships between environmental conditions and plants.
Lesson Learning Objectives
Students will be able to:- Apply findings from each week's lesson to make predictions and informed hypotheses about the next week's lesson.
- Keep a detailed laboratory notebook.
- Write and peer-edit the sections of a scientific paper, and collaboratively write an entire lab report in the form of a scientific research paper.
- Search for, find, and read scientific research papers.
- Work together as a team to conduct experiments.
- Connect findings and ideas from each week's lesson to get a broader understanding of how plants will respond to higher levels of CO2 (e.g., stomatal density, photosynthetic/respiratory rates, foliar protein concentrations, growth, and resource allocation).
Article Context
Course
Article Type
Course Level
Bloom's Cognitive Level
Vision and Change Core Competencies
Vision and Change Core Concepts
Class Type
Class Size
Audience
Lesson Length
Pedagogical Approaches
Principles of How People Learn
Assessment Type
INTRODUCTION
Origin and Rationale
In our previous introductory biology course sequence, students engaged in instructive, research-like lessons in hands-on laboratory courses. However, these lessons were relatively unrelated to one another, lacking obvious connections from lesson to lesson. We found that although students learned the scientific process and a wide array of biological topics, their learning remained at a superficial level. We think that this shortcoming reflected the structure of the course, which did not support students' ability to see how these lessons and their respective findings related to one another. Therefore, we redesigned our introductory biology curriculum into modules, containing a series of intentionally related lessons. This paper describes one module, which explores how plants will respond morphologically, physiologically, and biochemically to higher levels of CO2.
In this four-week module, student groups investigate plants and the connections among stomatal density (Supporting File S1: Stomatal Density Lesson), photosynthetic/respiratory rates (Supporting File S2: Photosynthesis and Respiration Lesson), foliar protein concentrations (Supporting File S3: Foliar Protein Lesson), and growth/resource allocation (Supporting File S4: Growth and Allocation Lesson). The stomatal imprint methodology in Lesson 1: Stomatal Density (Supporting File S1) is adapted from Grant and Vatnink 1998 (1). We specifically analyze how Brassica rapa (Fast Plants(R)) respond to low (400 ppm) and high (800 ppm) concentrations of atmospheric CO2. Elevated CO2 was chosen as our independent variable because it is an environmentally relevant and engaging topic as our students consider the environment of their futures. Brassica rapa was chosen because it is a fast-growing plant with a short life cycle (i.e., flower two weeks after planting); , it is an ideal model organism for short-term studies. Although we employed large growth chambers to control CO2 concentrations (Supporting Files S1: Stomatal Density Lesson; Supporting File S5: Supply List), the module is easily adaptable to other plant species and/or independent variables (e.g., light, nutrients, soil contaminants, genotypes, temperatures, biotic factors, etc.). Thus, the module can be adapted to instructor/student interests and available facilities/equipment.
Concentrations of atmospheric CO2 have dramatically risen in recent history (Figure 1) (2), primarily due to combustion of fossil fuels by humans (3). Pre-industrial levels of atmospheric CO2 were 280 ppm, but current levels are 401 ppm (Figure 1) (2). Many scientists are concerned about this increase because analysis of ancient gas trapped in ice cores indicates that CO2 concentrations were confined to between 180 and 280 ppm during the past 800,000 years (4,5). In fact, Earth has not experienced such consistently high concentrations of CO2 since the middle Miocene, approximately 15-20 million years ago (5). Since humans are predicated to continue to burn fossil fuels, CO2 concentrations are projected to reach 550 ppm by 2050 (6) and could be as high as 1000 ppm by the end of the century (7).

Figure 1. Trends in atmospheric carbon dioxide levels in recent history as recorded by NOAA (1). Used per the US Copyright Act section 105.
The direct impact of elevated concentrations of atmospheric CO2 on plant morphology, physiology, biochemistry, and growth has been an active area for research (8,9) and teaching (10,11). The focus on CO2 is not surprising considering that assimilation of CO2 by plants supports most heterotrophic life on Earth. Plants take in CO2 (an inorganic compound) from the atmosphere through their stomata via the process of photosynthesis. CO2 is then used to synthesize organic compounds, which are used by plants for maintenance, growth, and reproduction.
Lesson1: Stomatal response to elevated CO2 (Supporting File S1: Stomatal Density Lesson)
All plants do not respond to elevated atmospheric conditions of CO2 in the same way since like other living organisms, plant responses are influenced by both their genetic make-up and by the environment in which they live. Plants control the rate at which CO2 enters and H20 exits stomata by physiologically regulating the size of the stomatal opening or by morphologically modifying stomatal density during leaf expansion (e.g., 12-15). In our first lesson, we investigate the latter of these plant responses by analyzing the stomatal density of B. rapa grown in elevated CO2 compared to those grown in low CO2. Reducing water loss from transpiration through stomata is important for many plants to maintain cell turgor and growth. However, minimizing water loss through stomata (via transpiration) can also result in a decrease of CO2 concentrations inside the plant, which reduces photosynthetic rates and the ability of plants to make carbon-based molecules. Thus, stomata control is an important tradeoff in plants: they must allow sufficient CO2 into the plant to maintain growth, while minimizing loss of water. This means that for plant species that regulate stomatal conductance by altering stomatal density during leaf expansion, lowering stomatal density in high CO2 environments will generally increase fitness because plants can take in sufficient amounts of CO2 with minimal water loss (12,15).
Lesson 2: Photosynthetic and respiratory responses to elevated CO2 (Supporting File S2: Photosynthesis and Respiration Lesson)
A common physiological response of plants to elevated CO2 is an increase in photosynthetic rates, which can fuel increased growth rates and biomass accumulation (8,14). According to a meta-analysis of many scientific studies, plants grown under elevated CO2 conditions exhibit an average of a 31% increase in photosynthetic rates compared to plants grown under ambient CO2 conditions (16). This general response of plants, however, is not universal as photosynthetic rates of plants to elevated CO2 can be depressed by low temperatures and low water availability (17). Additionally, the responsiveness of plant species to elevated CO2 is strongly regulated by the metabolic pathway used to bind CO2 during photosynthesis. Photosynthetic rates of C3 plants respond most positively to elevated CO2 while C4 plants respond to a lesser extent or not at all (14,18).
The processes of photosynthesis and respiration are connected in that sugars made by photosynthesis are used via respiration to provide energy for growth and maintenance. However, there is no clear consensus on how plant respiration responds to elevated CO2 concentrations as increases, decreases, and no change have been reported (19,20).
Lesson 3: Response of foliar protein concentrations to elevated CO2 (Supporting File S3: Foliar Protein Lesson)
A common response of plants to elevated CO2 concentrations is a reduction in nitrogen concentrations in plant tissues. Meta-analyses indicate average reductions in nitrogen concentrations across plant species by approximately 10-15% (8,21). Many mechanisms have been proposed to explain reduced nitrogen concentrations in plants grown in elevated CO2 conditions. Two likely mechanisms include (1) dilution of nitrogen by increased photosynthetic rates and synthesis of carbon-based molecules and (2) reduction in nitrogen uptake by roots (22). Protein concentrations are linked to nitrogen concentrations in plant tissues. Therefore, it is no surprise that tissues of major food crops express a reduction in protein concentrations of approximately 10-15% (23). Decreases in protein concentrations in leaves are largely due to reductions in Rubisco (rubulose-1,5-bisphosphate carboxylase/oxygenase), the enzyme that binds to CO2 in the chloroplasts of plants (14).
Lesson 4: Increased plant biomass under elevated CO2 (Supporting File S4: Growth and Allocation Lesson)
Increased photosynthetic rates and water use efficiency of plants growing in elevated CO2 conditions typically translates into increased growth rates and biomass accumulation. Meta-analyses suggest an increase of approximately 20-29% in total plant biomass and more than 30% increase in biomass of belowground tissues (8,14).
Brassica rapa (Fast Plants®): a model plant
Due to their rapid rate of maturation and small size, B. rapa are commonly utilized as model plants for investigations in elementary, high school, and college classrooms (24); . The topics of these investigations are broad, spanning several subdisciplines of plant biology. Classroom experiments on B. rapa have been published investigating genetics (25,26,27), plant physiology [nutrition (28,29), tropisms (28), photosynthesis (30)], ecology (31,32), plant development (33), and evolution (34). Previously published activities using B. rapa largely focus on a particular topic like Mendelian genetics or artificial selection, whereas our module allows students to investigate how an environmental factor affects several attributes and processes fundamental to plant growth and survival. Therefore, this module fills a need for a suite of undergraduate-level investigations about B. rapa in an environmentally relevant context.
In this module, we investigate how elevated concentrations of CO2 will affect stomatal density (Supporting File S1: Stomatal Density Lesson), photosynthesis (Supporting File S2: Photosynthesis and Respiration Lesson), respiration (Supporting File S2: Photosynthesis and Respiration Lesson), protein concentrations (Supporting File S3: Foliar Protein Lesson), growth (Supporting File S4: Growth and Allocation Lesson) and resource allocation (Supporting File S4:Growth and Allocation Lesson) in B. rapa. For our investigations, 400ppm (low) CO2 concentration was chosen to model current CO2 conditions. We used 800 ppm (high) CO2 concentrations because it is twice as high as current levels but still within the range of projected CO2 concentrations by the end of this century (7). In our module, all environmental parameters other than CO2 are held constant: the temperature in all chambers is 23°C; the relative humidity is held at 70%; and B. rapa plants are grown in continuous light (for more information on planting and growing conditions, see Supporting File S1: Stomatal Density Lesson). Thus, students can isolate the effects of CO2 on the plants.
This module was developed so students can synthesize results from each lesson to gain a better understanding about how plants function and grow. In addition, when students begin their research, we ask them to consider how elevated CO2 will ultimately impact plant fitness and the future existence of plant species. Not only is this an interesting biological question, it also has direct impact on humans, since human life depends on plants, which provide oxygen, food, clothing, shelter, and medicine.
INTENDED AUDIENCE
This module was developed for students in an introductory biology laboratory course at a 4-year college. However, each lesson could be adapted to general education or upper level-plant biology courses.
REQUIRED LEARNING TIME
This module requires four-weeks, during which students meet weekly for one, three-hour laboratory. Additionally, in our curriculum students attend twelve 50-minute lectures that focus on plant biology and are complementary to laboratory material presented in these lessons. Students could also attend a weekly one-hour period to work on data analysis and collaborative writing of scientific reports related to each week's lesson.
PRE-REQUISITE STUDENT KNOWLEDGE
Students are required to read the background material about general plant responses to CO2 (Supporting File S6: Pre-module Background Reading), as well as procedures associated with each week's lesson before they come to class (Supporting Files S1-S4: See the Introduction and Procedure Sections in each Lesson). This requirement familiarizes students with basic concepts, terms, and procedures. We require students to complete a pre-lesson assignment in their lab notebooks in which they record the title, lesson objective(s), working hypothesis, and summarized procedures to ensure they are prepared for the lesson.
PRE-REQUISITE TEACHER KNOWLEDGE
To teach these lessons, teachers need a basic understanding of plant biology, compound microscopes, and spectrophotometry, as well as the ability to grow or have access to plants for student use in the classroom. Teachers can receive basic training related to these topics on various online platforms (e.g., 35-38). Teachers should also be familiar with independent and paired samples t-tests if they plan for students to use statistics to analyze data.
SCIENTIFIC TEACHING THEMES
ACTIVE LEARNING
In Class:
In contrast to passive learning, active learning involves students directly engaging in activities that shift the responsibility of learning from the instructor to the students. In this module, students work in small groups (3-4 students) throughout the entire module. During collaboration, students actively work together as they build scientific skills. In addition, students participate in hands-on laboratory activities that foster problem-solving and promote the construction of new knowledge. Also, students are responsible for actively maintaining an accurate, up-to-date lab notebook for the duration of the module.
Outside of Class:
Students perform and interpret data analyses and work with their small groups. They contribute to their lab notebook before each week's lesson by preparing a pre-lab section. Students also work on collaborative writing with peer review outside of class.
ASSESSMENT
Assessments for these modules include data gathered from five sources: (1) laboratory notebooks, (2) written lab reports, (3) student evaluation of participation of group members (Supporting File S7: Group Member Evaluation Form), (4) assignments (optional Pre-Lesson Worksheet in Supporting File S3: Foliar Protein Lesson), and (5) optional quizzes (Supporting File S8: Example Quiz).
First, laboratory notebooks are informally spot-checked to make sure that students are actively recording their activities. In addition, these notebooks are formally evaluated according to a grading rubric (Supporting File S9: Lab Notebook Grading Rubric) that assesses students on several key criteria (for criteria, see Supporting File S9: Lab Notebook Grading Rubric). Over the course of the four labs, each criterion is assessed at least two times (for details, see Supporting File S9: Lab Notebook Grading Rubric).
Second, students submit collaboratively written lab reports that are in the style of a scientific paper. Students have opportunities to submit multiple drafts, requiring both self-revision and peer-revision. Reports are graded according to a rubric (Supporting File S10: Collaborative Report Instructions and Grading Rubric). All students in a group experience being the author of a section and an editor for different sections. The grading process and author/editor model can be modified as desired to work in any laboratory context (for details, see Supporting File S10: Collaborative Report Instructions and Grading Rubric).
Third, each student submits a self-assessment that characterizes their own participation in the group and participation levels of other group members using a standard form at the end of each module (Supporting File S7: Group Member Evaluation Form). Although research on the value of student self-assessments (SSAs) is mixed (39), there is evidence linking SSA to enhanced metacognition and learning outcomes (39) and increased student motivation (40). Fourth, assignments from specific lessons are sometimes used as formative assessments. For example, the foliar protein lab (Supporting File S3: Foliar Protein Lesson) includes an assignment that can be used as a formative assessment. Finally, quizzes can be periodically given after each lesson or at the end of the module to gauge student understanding (Supporting File S8: Example Quiz).
INCLUSIVE TEACHING
This module is designed to mimic a true research experience as students apply findings from each week's lesson to inform hypotheses for the following weeks' lessons. By accurately representing the nature of science and scientific knowledge, these lessons promote problem-solving and curiosity as opposed to rote memorization. In doing so, students of all backgrounds find intellectual substance through active learning and have a chance to grow in their understanding of the nature of science, regardless of prior background.
In addition, students work in small, collaborative groups throughout the module. In these groups, students leverage each other's strengths and knowledge as they make predictions, test those predictions, gather evidence, analyze data, and communicate results. Furthermore, the module includes a breadth of activities including manipulation of live plants, conducting primary literature research, discussing/debating results, and co-writing/peer-editing the final lab report. Activities such as these that utilize multiple representations and engage students in multimodal experiences to promote inclusive learning for all races and ethnicities (41).
LESSON PLAN
BRASSICA RAPA GROWING CONDITIONS
We purchase B. rapa seeds from Carolina Biological, although any standard biological supply company typically carries the variety we use (Supporting File S5: Supply List). Standard B. rapa Fast Plants® seeds should be planted 10-14 days before the first lesson (Table 1). We plant seeds in accordance with the Fast Plants® Quad Growing System (42) with the exception that we use four pellets of Brassica fertilizer instead of three pellets. We have found that increasing the fertility improves plant vigor under our growing conditions.
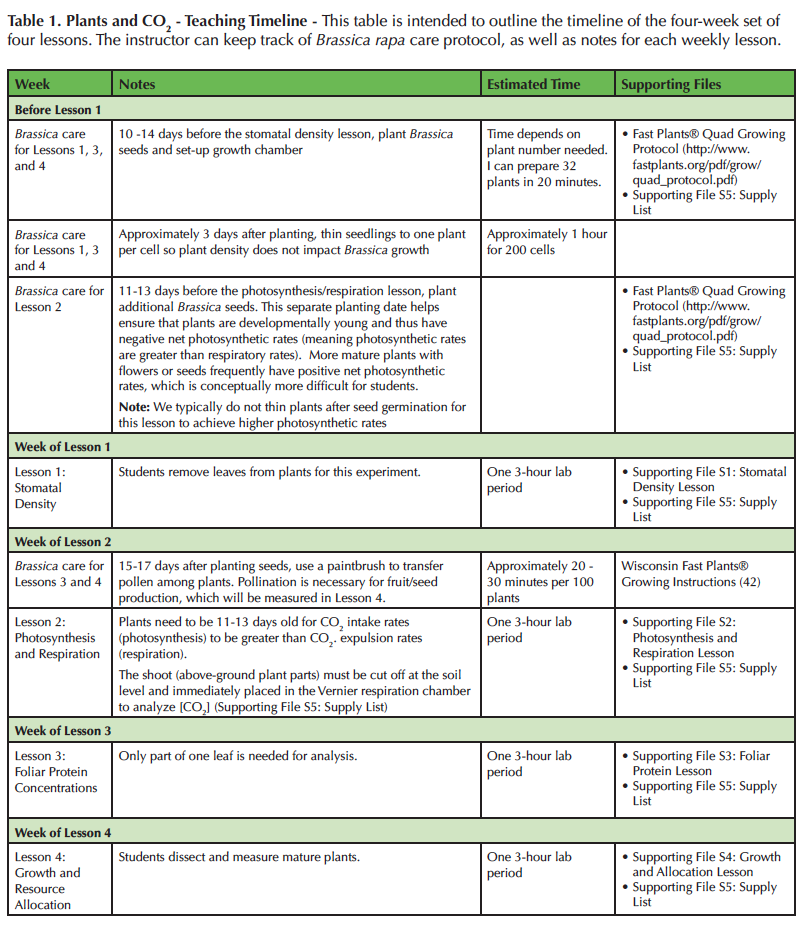
Table 1. Plants and CO2 - Teaching Timeline - This table is intended to outline the timeline of the four-week set of four lessons. The instructor can keep track of Brassica rapa care protocol, as well as notes for each weekly lesson.
Once planted, B. rapa should be grown in growth chambers with a temperature of 23°C, relative humidity of 70%, and continuous light. We position the tops of the plants at approximately 10 cm below 23 Watt, compact, fluorescent lights. To test the impact of elevated atmospheric CO2, half of the plants should be grown in 400 ± 50 ppm of CO2 and the other plants at 800 ± 50 ppm of CO2. The soil and plants stay relatively moist using the wicking mats as instructed by the Fast Plants® Growing System, but we additionally water cells every two to three days if they appear dry to maintain plant vigor. This additional watering is especially important during the germination period. Approximately three days after planting, we thin plants to one per cell (Table 1).
Before class begins, determine how many total plants are needed, but plan to grow extras to account for plant mortality. For Lesson 1 (Supporting File S1: Stomatal Density Lesson), each student analyzes two leaves: one leaf from a plant grown in high CO2 and one leaf from a plant grown in low CO2. If the plant has four true leaves (not cotyledons), then two plants are sufficient for a group of three to four students. After the leaves are removed from the plant, the plants are discarded because they no longer have enough leaves to survive. Similarly, two to four plants are needed per group for Lesson 2 (Supporting File S2: Photosynthesis and Respiration Lesson). For Lesson 2, the aboveground parts of the plants are excised from the roots to measure photosynthetic and respiratory rates, and thus the plants cannot be used again. For Lesson 3 (Supporting File S3: Foliar Protein Lesson), only one plant grown in high concentrations of CO2 and one plant grown in low concentrations of CO2 are needed per student group. Only one leaf per plant is analyzed, so the plants can be used again for Lesson 4 (Supporting File S4: Growth and Allocation Lesson) as long as each plant is returned to the correct CO2 concentration. See Table 2 for a breakdown of how many plants are typically grown for 50 groups of four students. The total number of plants needed to conduct all the lessons may seem overwhelming, but it is easy using Fast Plants®. Each tub grows 32 plants and only takes 32 cm x 20 cm of surface space! For example, we grow over 700 plants in four growth chambers.
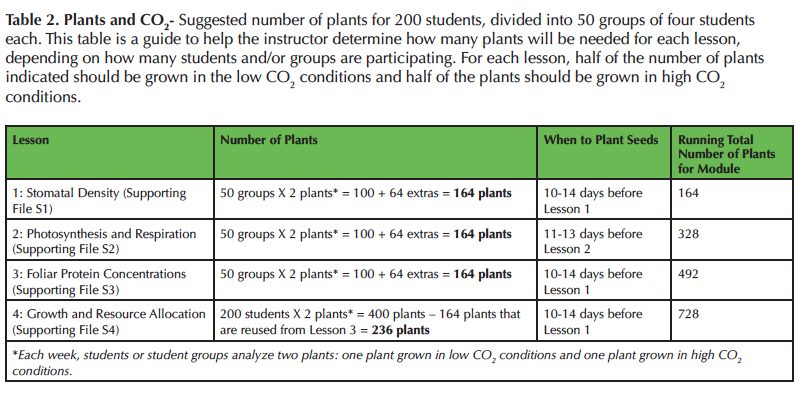
Table 2. Plants and CO2 - Suggested number of plants for 200 students, divided into 50 groups of four students each. This table is a guide to help the instructor determine how many plants will be needed for each lesson, depending on how many students and/or groups are participating. For each lesson, half of the number of plants indicated should be grown in the low CO2 conditions and half of the plants should be grown in high CO2 conditions.
INSERT TABLE 1 HERE
OVERVIEW OF PLANT MODULE
The plant module is designed to answer the following question, "How will plants respond to higher concentrations of CO2?" (Supporting Files S1-S4). The first lesson (Supporting File S1: Stomatal Density Lesson) allows students to visualize and count stomata on the underside of leaves using compound microscopes. Each student analyzes two leaves, one from a plant grown in 400 ppm of atmospheric CO2 and one from a plant grown in 800 ppm of atmospheric CO2. Then, students calculate stomatal density for each plant and enter their data into the course database. Students statistically analyze course results using an independent samples t-test. Based on their findings, students predict how CO2 concentrations will impact photosynthetic rates in Lesson 2.
In Lesson 2 (Supporting File S2: Photosynthesis and Respiration Lesson) students measure photosynthetic and respiratory rates of B. rapa in ambient conditions of CO2, after being grown under high or low CO2 concentrations. Plants are cut at the soil level so that their mass can be measured. Plants are then placed into a chamber connected to a CO2 gas sensor that measures CO2 concentrations in the chamber. The chambers with the cut plants are placed under a fluorescent lamp, and CO2 concentrations in the chamber are measured for 15 minutes. Next, the chamber is wrapped in aluminum foil, and plants acclimate to the darkness for a 5-minute period. CO2 concentrations are again measured in the chamber for 15 minutes. Students use the slope of the regression line between CO2 and time to estimate net photosynthetic and respiratory rates (ppm/min). Photosynthetic rates and respiratory rates are then standardized on a per-mg basis [(ppm/min)/mg]. As in Lesson 1, students enter data into a course database and statistically analyze the results.
The third lesson (Supporting File S3: Foliar Protein Lesson) allows students to measure water-soluble protein concentrations from the leaves of B. rapa grown in the high and low atmospheric CO2 concentrations. In this lesson, we assume the soluble protein present is predominantly comprised of photosynthetic enzymes like Rubisco, which binds CO2 from the atmosphere. Protein is extracted from leaves and combined with Bio-Rad protein assay dye. The concentration of the protein is then estimated using a spectrophotometer and a bovine serum albumin standard curve. As in the previous lessons, students enter data into a course database and statistically analyze the results.
The fourth and final lesson (Supporting File S4: Growth and Allocation Lesson) enables students to analyze growth and patterns of resource allocation of B. rapa grown in the different CO2 concentrations. Each student analyzes one plant from each of the CO2 treatments. Plants are removed from their cells, and roots are submerged in a tube of water and swirled around until the soil is removed. Students then measure total plant mass and partial plant mass of various other parts (e.g., root, shoot, and fruit). In addition, large seedpods are removed and dissected to count the number of mature, viable seeds. Once data from these variables are gathered, students calculate the root:shoot ratio and reproductive:vegetative ratio. These ratios indicate if the plant allocated photosynthate (i.e., carbon-based compounds) differently to plant structures. As before, students enter data into a course database and statistically analyze the results.
Surprisingly, we have found that B. rapa are unresponsive to elevated CO2 for all responses measured (Table 3), which does not support the majority of published research findings (e.g., 8,12,14,15,16,23). We use this opportunity to discuss the importance of anomalous and negative results. Most students assume that future elevated CO2 conditions will be beneficial for plants. However, after this module students realize that responses can be species specific. We then brainstorm hypotheses about why B. rapa may not follow the general trend of other C3 plants. One common hypothesis is that B. rapa (Fast Plants®) have been bred to grow at very high rates and that they may have reached a physiological maximum and cannot photosynthesize or grow at faster rates. Recently, we have also begun to explore different species (i.e., peppers) in our courses that do respond to elevated CO2 (43). Then we conduct small and large group discussions comparing results across species and brainstorm why some species respond to elevated CO2 while others do not.
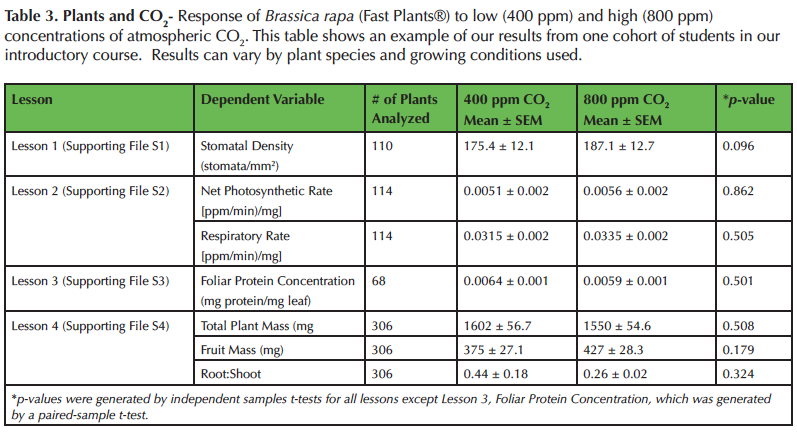
Table 3. Plants and CO2- Response of Brassica rapa (Fast Plants®) to low (400 ppm) and high (800 ppm) concentrations of atmospheric CO2. This table shows an example of our results from one cohort of students in our introductory course. Results can vary by plant species and growing conditions used.
MISCELLANEOUS: DATA FILE, STATISTICS, LAB NOTEBOOKS & WRITING
This module was designed for introductory-level biology students who want to be biology majors and/or want to pursue professional, health-related fields. In our inquiry-based labs for introductory biology, we emphasize the importance of supporting results with statistical evidence (i.e., claim - evidence - reasoning). Therefore, students use t-tests every week during this module to analyze data and interpret the results. To gather course data, we create a course database in which students across all sections enter their data. Then, the data are checked for errors and extreme outliers (two orders of magnitude or more) before the final data set is posted for data analysis.
In our introductory biology lab course, students keep a lab notebook with the following required information for each lesson: date, experiment title, group members' names, purpose of experiment, working hypothesis, data, calculations, statistical hypotheses (H0 and Ha), t-test output, figures and tables, results, and conclusions. At the end of the module, we assess the lab notebook using a grading rubric that consists of selected items from the required list assigned to each week's lab (i.e., "spot check"; Supporting File S9: Lab Notebook Grading Rubric). This approach allows us to give feedback to students as they learn these skills without grading the entire lab notebook.
In addition, students gain experience in scientific writing and peer-reviewing in this module by authoring a report in the style of a scientific journal. The writing assignments are designed to simulate how a group of collaborators might write and edit each other's work. During the first three lessons, a different section of a scientific paper (e.g., methods, results, etc.) is emphasized and discussed so that students can learn about that particular section. Students take turns writing and peer-reviewing these sections of the report until week four. At this point, students collaborate to make a final (and complete) report. In the final report, students divide the paper into sections, and each student serves as the lead author on at least one section. All students in the group also serve as editors of the other sections of the report. We feel this strategy helps ensure that everyone is involved in the writing process. Peer editors provide feedback using the comments feature in word processing software (e.g., Microsoft Word, Google Docs). Peer edits are retained, printed, and graded along with the final version of each section and completed report. All graded items are graded according to grading rubrics (Supporting File S10: Collaborative Report Instructions and Rubric).
Table 2 and 3 here
TEACHING DISCUSSION
These four lessons were designed to help students understand how anatomical (stomata), physiological (photosynthesis/respiration), and biochemical attributes (protein concentration) of plants interact and allow plants to survive, grow, and reproduce. We felt that investigating the impact of atmospheric concentrations of CO2 on plants provided an environmental context that many students would find relevant and relatable to societal concerns. In addition to content knowledge, this module was designed to develop students' basic scientific skills through authentic laboratory experiences. Specific skills that were emphasized included keeping lab notebooks, performing statistical analysis, writing scientific papers, and group collaboration.
STUDENT REACTIONS TO LESSONS AND ASSESSMENTS
Our previous experiences indicate that students do not typically enjoy keeping a lab notebook as it takes considerable time. However, we felt this was an important scientific skill as professional labs require scientists to keep detailed records and most health-related professions require accurate and detailed record-keeping. Therefore, to minimize student time on lab notebooks out of class, we emphasized that students get as much done as possible during lab and required that students had recorded all their group’s data in their lab notebooks before they could leave lab. We have found that many students were not effectively using class time and did not record data as they worked because they wanted their lab notebooks to be “perfectly organized,” which is unrealistic and not an authentic scientific practice.
Additionally, students had mixed reactions to statistical analysis of data. At the beginning of the course, many students said that statistical analysis was confusing. However, we typically observed that most students easily conducted and interpreted t-tests after a few weeks of practice. Some students appreciated the use of probability and analytical evidence in interpreting and providing evidence for their results. Moreover, students who have gone on to graduate school or research have told us that they were better prepared than their peers to conduct research and succeed in graduate-level courses in part because of their statistical skills.
As part of our assessment, we solicited feedback from teaching assistants (TAs) as well as students. Our TAs were undergraduates who had taken the course previously. Feedback from TAs indicated the plant module effectively helped students achieve the desired learning goals. TAs noted that the related lessons gave students a broader understanding of plants, and according to one TA, brought "a simple topic... to life before the students' eyes." TAs also indicated the modular approach facilitated students' making conceptual connections. For example, students learned to evaluate B. rapa's resource allocation based on varying CO2 levels and previously measured attributes (e.g., stomatal density) and processes (e.g., net photosynthesis). One TA stated, "I think students engaged in the growth and resource allocation lab... some were asking me good questions about Brassica rapa."
TAs generally found that students had positive attitudes about this module. For example, TAs reported that students were "fascinated with how something so small [stomata] could be found and evaluated during lab." Students also appreciated the practical nature of the lab as they investigated how "simple plants are alive and constantly doing important chemical reactions." Furthermore, TAs stated that students were engaged because of the environmental relevance of the topic. According to one TA, students "evaluate the effect of increased levels of carbon dioxide, which is becoming a world problem. [Students] get to help research the effects." Students seemed to appreciate the opportunity to do research on an important environmental topic.
TAs advised that the final report is an important component. Students learned to ask questions, formulate hypotheses, gather/analyze data, predict outcomes, and display results. However, students also had to think deeply about these components by writing and reviewing the final report. One TA explained how writing "requires students to understand." Moreover, at the end of the module, students will be able to connect findings and ideas from all lessons to get a bigger picture of how plants work.
ADAPTABILITY OF LESSONS
While this module works well in our current setup, we recognize the ability to grow plants in elevated CO2 conditions will likely be limited at many institutions. Therefore, analyzing different independent variables (e.g., soil fertility, light intensity, soil contaminants, etc.) in your classrooms, guided by both interest and available equipment, is encouraged. Similarly, we use Brassica rapa Fast Plants® for our research because they mature from seed to flowering in about two weeks and take very little space. However, any small, relatively fast-growing plant would work, if the space and resources needed to grow the plant species are available. For instance, following student interest, we recently substituted B. rapa with a hot pepper plant (Capsicum annuum 'Poinsettia') in this module and had an interesting module of experiments where we could compare responses of a different species to responses of B. rapa.
In our course, students collaborate in a group to write a group lab report. However, depending on course goals or instructor preferences, each student could instead write an individual lab report. One model we have used in the past is for one student per group to choose one of the four lessons to write his/her report. This model also works well because students have input about what they will write. Additionally, the papers are then turned in over a four-week period, helping the instructor keep up with grading.
Finally, each lesson could easily be taught in isolation. The ability for students to complete and understand each lesson is not contingent on completing any other lesson beforehand.
SUPPORTING FILES
- S1. Plants and CO2-Lesson 1: Stomatal Morphology and Density: Student Lab Manual Pages (.docx)
- S2. Plants and CO2-Lesson 2: Photosynthesis and Respiration: Student Lab Manual Pages (.docx)
- S3. Plants and CO2-Lesson 3: Foliar Protein Concentrations: Student Lab Manual Pages (.docx)
- S4. Plants and CO2-Lesson 4: Growth and Resource Allocation: Student Lab Manual Pages (.docx)
- S5.Plants and CO2-Supply List per Lesson (.docx)
- S6: Plants and CO2-Pre-module Background Reading: Plant Responses to CO2 (.docx)
- S7. Plants and CO2-Group Member Evaluation Form (.docx)
- S8. Plants and CO2-Example Quiz (.docx)
- S9. Plants and CO2-Lab Notebook Sample Grading Rubric (.docx)
- S10. Plants and CO2-Collaborative Report Instructions and Grading Rubric (.docx)
References
- Grant B, Vatnick I. 1998. A multi-week inquiry for an undergraduate introductory biology laboratory: investigating correlation between environmental variables and leaf stomata density. J. Coll. Sci. Teach. 28:109-112.
- U.S. Department of Commerce. 2017. Trends in atmospheric carbon dioxide, URL https://www.esrl.noaa.gov/gmd/ccgg/trends. Accessed 01/16/2017.
- Le Quéré C, Raupach M, Canadell J, Marland G. 2009. Trends in the sources and sinks of carbon dioxide. Nat. Geosci. 2:831-836.
- Lüthi D, Le Floch M, Bereiter B, Blunier T, Barnola JM, et al. 2008. High-resolution carbon dioxide concentration record 650,000-800,000 years before present. Nature 453:379-382.
- Tripati A, Roberts C, Eagle R. 2009. Coupling of CO2 and ice sheet stability over major climate transitions of the last 20 million years. Science 326:1394-1397.
- IPCC. 2001. Climate change 2001: The scientific basis. In Houghton JT, Ding Y, Griggs DJ, Noguer M, van der Linden PJ, Dai X, Maskell K, Johnson CA (eds), Contribution of working group I to the third assessment report of the intergovernmental panel on climate change. Cambridge University Press, Cambridge, United Kingdom and New York, NY, USA, 881 pp.
- IPCC. 2007: Climate Change 2007: The physical science basis. In Solomon S, Qin D, Manning M, Chen Z, Marquis M, Averyt KB, Tignor M, Miller HL (eds), Contribution of Working Group I to the Fourth Assessment Report of the Intergovernmental Panel on Climate Change Cambridge University Press, Cambridge, United Kingdom and New York, NY, USA, 996 pp.
- Curtis P, Wang X. 1998. A meta-analysis of elevated CO2 effects on woody plant mass, form, and physiology. Oecologia 113:299-313.
- Lindroth R. 2010. Impacts of elevated atmospheric CO2 and O3 on forests: phytochemistry, trophic interactions, and ecosystem dynamics. J. Chem. Ecol. 36:2-21.
- Williams, MA, and Wassenberg, D. 2017. Promoting climate change literacy for non-majors: Implementation of an atmospheric carbon dioxide modeling activity as an inquiry-based classroom activity. CourseSource. https://doi.org/10.24918/cs.2017.12
- Smith, MK, Toth, ES, Borges, K, Dastoor, F, Johnston, J, Jones, EH, Nelson, PR, Page, J, Pelletreau, K, Prentiss, N, Roe, JL, Staples, J, Summers, M, Trenckmann, E, Vinson, E. 2018. Using place-based economically relevant organisms to Improve student understanding of the roles of carbon dioxide, sunlight, and nutrients in photosynthetic organisms. CourseSource. https://doi.org/10.24918/cs.2018.1
- Woodward FI. 1987. Stomatal numbers are sensitive to increases in CO2 from preindustrial levels. Nature 327:617-618.
- Royer DL, Wing SL, Beerling DJ, Jolley DW, Koch PL, Hickey LJ, Berner RA. 2001. Paleobotanical evidence for near present-day levels of atmospheric CO2 during part of the Tertiary. Science 292:2310-2313.
- Ainsworth EA, Long SP. 2005. What have we learned from 15 years of free-air CO2 enrichment (FACE)? A meta-analytic review of the responses of photosynthesis, canopy properties and plant production to rising CO2. New Phytol. 165:351-372.
- Haworth M, Elliott-Kingston C, McElwain JC. 2013. Co-ordination of physiological and morphological responses of stomata to elevated [CO2] in vascular plants. Oecologia 171:71-82.
- Ainsworth EA, Rogers A. 2007. The response of photosynthesis and stomatal conductance to rising [CO2]: mechanisms and environmental interactions. Plant cell Environ. 30:258-270.
- Morison JIL, Lawlor DW. 1999. Interactions between increasing CO2 concentration and temperature on plant growth. Plant, Cell, Environ. 22:659-682.
- Leakey ADB, Ainsworth EA, Bernacchi CJ, Rogers A, Long SP, Ort DR. 2009. Elevated CO2 effects on plant carbon, nitrogen, and water relations: six important lessons from FACE. J. Exp. Bot. 60:2859-2876.
- Wang XZ, Curtis P. 2002. A meta-analytical test of elevated CO2 effects on plant respiration. Plant Ecol. 161:251-261.
- Gonzalez-Meler MA, Taneva L, Trueman RJ. 2004. Plant respiration and elevated atmospheric CO2 concentration: cellular responses and global significance. Ann. Bot. 94:647-656.
- Cotrufo M, Ineson P, Scott A. 1998. Elevated CO2 reduces the nitrogen concentration of plant tissues. Global Change Biol. 4:43-54.
- Taub DR, Wang X. 2008. Why are nitrogen concentrations in plant tissues lower under elevated CO2? A critical examination of the hypotheses. J. Integr. Plant Biol. 50:1365-1374.
- Taub DR, Miller B, Allen H. 2008. Effects of elevated CO2 on the protein concentration of food crops: a meta-analysis. Global Change Biol. 14:565-575.
- Wisconsin Fast Plants. 2016. Lessons and Investigations. URL https://fastplants.org/lessons/. Accessed 03/22/2017.
- Batzli JM, Smith AR, Williams PH, McGee SA, Dosa K, Pfammatter J. 2014. Beyond Punnett squares: student word association and explanation of phenotypic variation through an integrative quantitative genetics unit investigating anthocyanin inheritance and expression in Brassica rapa Fast Plants. CBE Life Sci. Educ. 13:410-424.
- Wendell DL, Pickard D. 2007. Teaching human genetics with mustard: rapid cycling Brassica rapa (Fast Plants type) as a model for human genetics in the classroom laboratory. CBE Life Sci. Educ. 6:179-185.
- Wisconsin Fast Plants. Investigating Mendelian genetics with Wisconsin Fast Plants. URL https://fastplants.org/pdf/activities/WFPgenetics-06web.pdf. Accessed 03/22/2017.
- Wisconsin Fast Plants. Investigating plant physiology with Wisconsin Fast Plants. URL https://fastplants.org/pdf/activities/WFPphysiology-06web.pdf. Accessed 03/22/2017.
- Lee CA. 2003. A learning cycle inquiry into plant nutrition. Am. Bio. Teacher 65:136-141.
- Wisconsin Fast Plants. Exploring photosynthesis with Fast Plants. URL https://fastplants.org/resources/digital_library/index.php?P=FullRecord&ResourceId=10. Accessed 03/22/2017.
- Barko VA, Burke BA, Gibson DJ, Middleton BA. 2004. Seedling growth of Wisconsin Fast Plants (Brassica rapa) in field environments. TIEE 1:1-15.
- Wisconsin Fast Plants. 1987. Bees and Brassicas: a partnership in survival. URL https://fastplants.org/pdf/activities/bees_brassicas.pdf. Accessed 3/2/2017.
- Wisconsin Fast Plants. 1990. Double fertilization and post-fertilization events: measuring. URL https://fastplants.org/resources/digital_library/index.php?P=FullRecord&ResourceId=42. Accessed 3/22/2017.
- Lauffer D, Fall BA. 2000. Evolution by artificial selection and unraveling the mysteries of hairy's inheritance. p 147-179. In Karcher SJ (Ed), Tested studies for laboratory teaching, Volume 21. Proceedings of the 21st Workshop/Conference of the Association for Biology Laboratory Education (ABLE), 509 pages.
- Chamovitz, D. 2018. Understanding plants - Part 1: What a plant knows. URL https://www.coursera.org/learn/plantknows. Accessed 5/24/18.
- Chamovitz, D. 2018. Understanding plants - Part 2: Fundamentals of plant biology. URL https://www.coursera.org/learn/plant-biology. Accessed 5/24/18.
- Introduction to microscopy. 2018. URL http://www.open.edu/openlearn/science-maths-technology/science/biology/introduction-microscopy/content-section-0?active-tab=description-tab. Accessed 5/24/18.
- Spectrophotometry introduction. 2018. URL https://www.khanacademy.org/science/chemistry/chem-kinetics/spectrophotometry-tutorial/v/spectrophotometry-introduction. Accessed 5/24/18.
- Pandero E, Brown GTL, Strijbos J. 2016. The future of student self-assessment: a review of known unknowns and potential directions. Educ. Psychol. Rev. 28:803-830.
- McMillan JH, Hearn J. 2008. Student self-assessment: the key to stronger student motivation and higher achievement. Educational Horizons 87:40-49.
- NGSS Lead States. 2013. Next Generation Science Standards: For States, By States. Washington, DC: The National Academies Press.
- Wisconsin Fast Plants. Wisconsin Fast Plants(R) Growing Instructions. https://fastplants.org/pdf/growing_instructions.pdf. Accessed 2/2/2018.
- Muilenburg VL, Hertel L, Winnett-Murray K. In press. Comparing two model plants for use in an introductory biology course: Wisconsin Fast Plants(R) and Poinsettia hot peppers. Poster. Assoc. for Biol. Lab. Educ. (ABLE).
Article Files
Login to access supporting documents
A flexible, multi-week approach to plant biology - How will plants respond to higher levels of CO2?(PDF | 260 KB)
S1. Plants and CO2-Lesson 1-Stomatal Morphology and Density - Student Lab Manual Pages.docx(DOCX | 3 MB)
S2. Plants and CO2Lesson 2 - Photosynthesis and Respiration - Student Lab Manual Pages.docx(DOCX | 2 MB)
S3. Plants and CO2-Lesson 3 - Foliar Protein Concentrations - Student Lab Manual Pages.docx(DOCX | 228 KB)
S4. Plants and CO2-Lesson 4 - Growth and Resource Allocation - Student Lab Manual Pages.docx(DOCX | 30 KB)
S5. Plants and CO2-List of Supplies per Lesson.docx(DOCX | 23 KB)
S6. Plants and CO2-Pre-module Background Reading - Plant Responses to CO2.docx(DOCX | 1 MB)
S7. Plants and CO2-Group Member Evaluation Form.docx(DOCX | 15 KB)
S8. Plants and CO2-Example Quiz.docx(DOCX | 252 KB)
S9. Plants and CO2-Lab Notebook Sample Grading Rubric.docx(DOCX | 21 KB)
S10. Plants and CO2-Collaborative Report Instructions and Grading Rubric.docx(DOCX | 256 KB)
- License terms
Comments
Comments
There are no comments on this resource.