What do Bone and Silly Putty® have in Common?: A Lesson on Bone Viscoelasticity
Published online:
Abstract
Without the use of real-life examples and models, actively instructing and engaging students in complex physiology topics related to bone biomechanics can be challenging. In our large-enrollment Human Physiology & Anatomy courses at the University of Connecticut, the skeletal system is the first organ system that we cover in depth, rendering even more important our establishment of (and emphasis on) how fascinating--and directly applicable to everyday life--the anatomical features and physiological properties of organs can be. Because our lecture courses are supplemented with 2 hours per week of anatomy-intensive laboratory investigations, we tend to focus heavily on physiology concepts during lecture. For the skeletal system, we consider stress-strain curves in the context of bone biomechanics, including the materials-science concepts of elasticity, plasticity, and viscoelasticity, and the more generalizable concepts of hysteresis and anisotropy. Hoping to provide a fun, entertaining, and real-world perspective on these topics, we used Silly Putty® as an inexpensive, familiar, and readily-available model of some of these bone properties. This lesson allows students to get engaged in, and familiarized with, biomechanical concepts through demonstration of this well-known play material's properties, as a concrete reference point. Coupling the lesson with active questioning and think-pair-share activities allows students to develop skills in data interpretation and to apply previously-acquired knowledge bases to a novel situation. In this lesson, we provide instructors with a template for re-creating this demonstration, which can be accompanied by active-learning strategies and resources that promote development of data-interpretation and problem-solving skills in students.
This article has an accompanying Science Behind the Lesson article: "A Short Bone Biomechanics Primer: Background for a Lesson on Bone Viscoelasticity."
Citation
Redden, J.M., Tzingounis, A.V. and Tanner, G.R. 2019. What do Bone and Silly Putty® have in Common?: A Lesson on Bone Viscoelasticity. CourseSource. https://doi.org/10.24918/cs.2019.12Lesson Learning Goals
- Students will understand the principles of bone biomechanics.
- Students will understand how the structure of bones complements their functions.
Lesson Learning Objectives
- Students will be able to explain how the anatomical structure of long bones relates to their function.
- Students will be able to define viscoelasticity, hysteresis, anisotropy, stiffness, strength, ductility, and toughness.
- Students will be able to identify the elastic and plastic regions of a stress-strain curve. They will be able to correlate each phase of the stress-strain curve with physical changes to bone.
- Students will be able to predict how a bone would respond to changes in the magnitude of an applied force, and to variations in the speed or angle at which a force is applied.
- Students will be able to determine the reason(s) why bone injuries occur more frequently during athletic events than during normal everyday use.
Article Context
Course
Article Type
Course Level
Bloom's Cognitive Level
Vision and Change Core Competencies
Vision and Change Core Concepts
Class Type
Class Size
Audience
Lesson Length
Pedagogical Approaches
Principles of How People Learn
Assessment Type
INTRODUCTION
BACKGROUND
Historically, in our Human Physiology & Anatomy courses, the skeletal system is the first organ system that we cover in depth in the courses' first term, typically following 2 or 3 lectures of introductory material regarding membrane transport, homeostasis and feedback loops, tissue classification, and types of epithelia. While very accessible to students as an entry point into learning about systems of the body, the study of the bones as organs can be quite dry for instructor and student alike if the skeletal system is considered primarily from a gross-anatomical perspective.
Because of the design of our courses, wherein the lecture component is supplemented with 2 hours per week of hands-on laboratory work that is very anatomy-intensive, we focus during lecture on the more-complex aspects of the relevant physiology for each topic. In so doing, we aim to strike a balance in our presentation of the material to make it both interesting and intellectually challenging. Therefore, while we aspire to work on fostering deeper understanding of physiological concepts as well as on building student skills in data analysis and interpretation, we also strive to find ways to make our discussions of physiology accessible, engaging, and even--to the degree possible--entertaining. Including discussions of everyday applications of the physiological topics that we cover serves to introduce a real-life relevance to these topics, which directly addresses the goals of accessibility and greater student engagement in the course material.
To apply a more physiological bent to our discussion of the bones, we have focused one aspect of our skeletal-system lectures on the biomechanical properties of the bones. Many bone biomechanical properties can be analyzed using stress-strain curves (see References 1 and 2 and Figure 1), a type of force-displacement curve that plots measured stress (pressure) on a material against applied strain (deformation). Such curves typically feature straight, linear elastic regions for their initial portions, which lead into non-linear plastic regions that tail off to an end point on the curve, representing material failure.

Figure 1. Representative example stress-strain curves for a generic material. (A) The elastic region of the curve shows a linear stress-strain relationship. (B) The full curve shows the elastic region, yield point, plastic region, and failure point for this generalized material.
We can then consider how these biomechanical properties relate to our everyday lives as organisms, each possessing a bony endoskeleton that is subjected to various stresses and strains on a daily basis. Among the relevant mechanical properties of bone as a bio-material are: elasticity, which is the ability of a material to resist deformation due to stress and to return to the original size and shape upon offloading; plasticity, which is non-reversible deformation with stress; hysteresis, which is a recent-history dependence of a material's behavior; anisotropy, which is a directional dependence of behavior; and viscoelasticity, which is a dependence of behavior on the rate of loading. This last property--viscoelasticity--is the topic of focus for this Lesson. (For more information on biomechanical terminology and stress-strain curves, please see Supporting Material S1. Bone Viscoelasticity: Bone Biomechanics Primer and the accompanying Science Behind the Lesson article: "A Short Bone Biomechanics Primer: Background for a Lesson on Bone Viscoelasticity," both of which include detailed definitions and explanations of terms and concepts.)
In the second term of our course, we return to force-displacement curves several times, most notably in our discussion of the vasculature: blood vessel compliance curves plotting how veins and arteries behave differently with respect to their volume changes (consequent to strain on the surrounding tissue) in response to pressure changes (i.e. stress on the tissue). Discussing bone biomechanics in depth in the first term of our course provides students with an early concrete example of the construction and interpretation of biological force-displacement curves that students can later apply to many other physiologically-relevant contexts.
For our own specific purposes, lessons such as these also help to establish for our students, very early on in the academic year, the idea that the course will not center exclusively on rote memorization of terminology, facts, and concepts, but will require their frequent application as well. We also emphasize that the course will regularly involve analysis and interpretation of physiological data, including discussions of how physiological experiments are designed and executed.
OVERVIEW
This lesson primarily covers viscoelasticity, a time- or rate-dependent property of bone wherein its deformational response to stress (i.e., its strain) is dependent upon the rate of loading (see Reference 3 and Figure 2). Bone, like other viscoelastic materials, will exhibit increased stiffness and yield strength in response to increased rates of loading (3). However, as rates of loading increase, bone becomes less tough and more brittle, failing (i.e. buckling or breaking) more readily under conditions of very high loading rates (3). In contrast, bone is more flexible and ductile, with greater toughness, at lower, everyday-use loading rates (3). These properties are evident from the family of stress-strain curves generated by applying different strain rates to actual samples of cortical bone (the outermost, dense portions of long bones) and measuring the resulting stresses (see Reference 3 and Figure 2).

Figure 2. A stylized representation of data from an experiment on bone viscoelasticity. The graph shows several stress-strain curves for cortical bone, generated at different loading rates, overlain on the same set of axes. Data adapted from Reference 3.
In principle, this entire lesson on viscoelasticity could be taught exclusively through the discussion and interpretation of the stress-strain curves graph alone (Figure 2). However, the whole concept of viscoelasticity can really be brought alive in dramatic fashion by the accompanying demonstration with Silly Putty®, which--in a manner analogous to the behavior of bone--will resist deformation better the harder it is stressed.
In our opinion, one of the most useful aspects of the lesson is the opportunity to provide students a live demonstration with an easily-manipulated physical model of bone. Additionally, it is very compelling to model a bio-mechanical property of bone with an everyday plaything that most people would never imagine to have anything remotely in common with bone. Setting the demonstration up as a question of what bone and Silly Putty® have in common poses a question that grabs student attention and which is readily answerable within less than 10 minutes. At the same time, the demonstration and discussion serve to reinforce concepts of bone biomechanics that have been previously covered, as well as to extend and deepen students' understanding of the principles of biomechanics with reference to bone properties.
INTENDED AUDIENCE
This lesson is intended for undergraduate majors in biological and biomedical sciences at a four-year college or university. This lesson was taught to second- and third-year majors in Physiology and Neurobiology (PNB) at the University of Connecticut, in their core introductory Enhanced Human Physiology and Anatomy lecture course (PNB 2274), which enrolls nearly 400 students. The lesson could be taught as presented herein; however, because we are including extensive supplemental materials (see Supporting Materials S1. Bone Viscoelasticity: Bone Biomechanics Primer; S2. Bone Viscoelasticity: Worksheet; S3. Bone Viscoelasticity: Bone Biomechanics Lecture Slides; and S4. Bone Viscoelasticity: Bone Biomechanics Assessment Questions) as well as a video demonstration (Supporting Material S5. Bone Viscoelasticity: Bone Viscoelasticity Lecture Recording), this module could also easily be directly delivered as an extension or honors-level assignment in introductory courses or courses targeted at non-science majors.
REQUIRED LEARNING TIME
This lesson is a portion of a larger lesson on bone biomechanics (see Supporting Materials S1. Bone Viscoelasticity: Bone Biomechanics Primer; S2. Bone Viscoelasticity: Worksheet; and S3. Bone Viscoelasticity: Bone Biomechanics Lecture Slides). It requires roughly ten minutes of time in lecture to present the demonstration along with a discussion of a graphical representation of measured viscoelastic properties of bone (see Figure 2 and Supporting Material S3. Bone Viscoelasticity: Bone Biomechanics Lecture Slides). Both the demonstration and the follow-up discussion of the family of stress-strain curves depicting viscoelasticity allow for plenty of review of previously-studied terminology and topics in bone biomechanics, such as elasticity, plasticity, and hysteresis. The discussion portion of the lesson poses questions probing students' depth of understanding of this newly-introduced concept of viscoelasticity. This latter aspect of the activity--a set of questions that can be posed as think-pair-share activities or clicker questions--can allow for in-the-moment assessment of students' ability to apply previously-established knowledge to novel situations.
PRE-REQUISITE STUDENT KNOWLEDGE
Students should already know the key terminology related to bone biomechanics (see Supporting Material S1. Bone Viscoelasticity: Bone Biomechanics Primer and the accompanying Science Behind the Lesson article: "A Short Bone Biomechanics Primer: Background for a Lesson on Bone Viscoelasticity"). Students should also be familiar with basic conceptual, qualitative interpretations of stress-strain curves. Students will have previously been exposed to graphs displaying single or multiple stress-strain curves on the same set of axes for a discussion of general biomechanics as well as a specific discussion of bone anisotropy (see Supporting Material S3. Bone Viscoelasticity: Bone Biomechanics Lecture Slides).
PRE-REQUISITE TEACHER KNOWLEDGE
Instructors should be very comfortable with the idea of force-displacement curves (e.g. Hooke's law for springs) and the use and application of stress-strain curves to biomechanics and/or materials science. Instructors wishing to incorporate this lesson into their courses who are not entirely familiar with these concepts can read the accompanying Science Behind the Lesson article ("A Short Bone Biomechanics Primer: Background for a Lesson on Bone Viscoelasticity") as an additional primer.
SCIENTIFIC TEACHING THEMES
ACTIVE LEARNING
Engaging students in active learning in a large lecture classroom presents many challenges, not least of which is the sheer number of students present. With an attention-grabbing demonstration such as this--with balls of Silly Putty® being actively stretched, crushed, thrown, and bounced around the lecture hall, there is a palpable uptick in interest and engagement in the classroom. Bone mechanics, like most engineering concepts, is often explained using dense vocabulary which is likely to be unfamiliar to most students. As such, students often struggle to find ways to incorporate the information delivered via lecturing. For this lesson, having the students complete the pre-class primer and/or worksheet (Supporting Material S1. Bone Viscoelasticity: Bone Biomechanics Primer, Supporting Material S2. Bone Viscoelasticity: Worksheet) places the responsibility for understanding the terminology that will be used during the demonstration on the students themselves. Depending on the resources available to the instructor, the beginning of class would be an ideal place to incorporate a formative assessment of the students' retention of these self-taught concepts using a classroom response system (e.g. clickers or polling software). For example, instructors could ask students to classify a list of everyday materials as being stiff (carpentry nails, golf balls, tin cups) or flexible (fingernails, kickballs, paper cups); or to rank a series of common materials (such as plastic bottles, aluminum foil, and polyethylene bags) in order of decreasing strength.
During the lesson itself, the suggested think-pair-share questions asking students to make comparisons between different points on the stress-strain curves (or between different conditions), facilitate peer and instructor discussion, which are the generally-accepted hallmarks of an active classroom. (See, for reference, slides 11-13 of Supporting Material S3. Bone Viscoelasticity: Bone Biomechanics Lecture Slides.) Moreover, the one-minute essay wrap-up question, relating bone fracture patterns back to the biomechanical concepts described in the lesson, create an opportunity for reflection and further in-class discussion. (For reference, see slide 15 of Supporting Material S3. Bone Viscoelasticity: Bone Biomechanics Lecture Slides.)
Depending on the size and setup of the classroom, and if time permits, the interpretation of fracture patterns could also be easily adapted into a jigsaw activity to further increase engagement and discussion within the classroom. For example, small groups of students could be assigned a specific fracture pattern to research, identify, and explain. Upon each group's reaching of a consensus, students from a given group join another group, and exchange ideas and explain their answer and rationale. This process continues until the class has reached a consensus on all fracture patterns.
ASSESSMENT
Learning is measured both in real time with student responses to questions posed by the instructor during the lecture and with questions on bone biomechanics on a multiple-choice test (Supporting Material S4. Bone Viscoelasticity: Bone Biomechanics Assessment Questions).
INCLUSIVE TEACHING
This lesson includes several visual elements, focusing primarily on a graphical analysis of the projected stress-strain curve and on the real-time manipulation of Silly Putty®. This manipulation also incorporates a kinesthetic aspect to the lesson, especially for those students who have the chance to handle the putty. Thus, this lesson may better attract the attention of students who preferentially approach learning through visual-spatial or bodily-kinesthetic means (4), as opposed to primarily through the more-traditional verbal-linguistic or logical-mathematical means.
This real-world experiential demonstration may also appeal more to students who might be located within the "converger" realm of David Kolb's learning styles, as laid out in his 1984 treatise on experiential learning (5). Such students fall closer to the "active experimentation" end on the processing dimension and the "abstract conceptualization" end on the perception dimension (5), giving them some of the qualities associated with engineers, whose work involves iterative modes of thinking conceptually and then instantiating and executing ideas in the real world to see if their ideas work in practice. Those students who do have the opportunity actually to handle the Silly Putty® material may be able to approach the lesson from the learning perspectives of either a "Pragmatist"--putting abstract concepts into real-world practice--and/or a "Reflector"--observing and then subsequently thinking about activities (6).
Though the students themselves, in a large lecture classroom, cannot all have the concrete tactile experience of directly handling the Silly Putty®, they are all in fact able to watch it being handled. Such observation can engage the mirror neuron system, which is thought to be essential for learning by imitation (for a review of this system, see Reference 7). Thus, the demonstration has the potential for also possibly tapping into other sensory modalities (e.g., touch), or cortically-mirrored motor planning to enrich the student experience of the lesson.
While the practice of specifically modifying teaching methodologies to match preferred student learning styles has come under recent scrutiny and skepticism (8,9), engaging students of different academic backgrounds and life experiences remains nevertheless as important as it is challenging, particularly when discussing dense material such as this. Therefore, framing the lesson within the context of athletics, and using familiar objects, can render the lesson less intimidating and make the content more universally accessible. We designed this activity so it may facilitate meaningful connections for the students, perhaps even including personal ones. Building such connections may result in improvements to the affective domain (10) of students' relationships to and perceptions or understanding of the coursework. Reciprocally, improvements in the affective domain have the potential to positively impact students' attitudes towards and personal attachment to STEM material. Being able to draw links between the course material and everyday-life applications helps students--especially from underrepresented minority groups--develop a greater sense of belonging in STEM fields (11), and thus enhances their experience in the classroom and the course, which may additionally have the beneficial effect of aiding in student retention.
LESSON PLAN
PRE-CLASS PREPARATION
For the instructor, it is essential to have a few containers of Silly Putty® or a similar such substance available for the lesson. It bears noting that the Silly Putty® should be bought fresh; it will dry out and lose its essential properties if stored for long times, even in humid environments. In smaller classrooms, of 30 students or fewer, three to five containers of putty may be enough for all students to have the opportunity to handle the material. For the students, it is strongly recommended that they will have read the primer on bone biomechanics prior to attending class (Supporting Material S1. Bone Viscoelasticity: Bone Biomechanics Primer). Additionally, it will be useful if the students will have attempted either the mini problem set accompanying the Primer, or the related worksheet-based matching exercise, or both activities (Supporting Material S2. Bone Viscoelasticity: Worksheet; and slide 6 of Supporting Material S3. Bone Viscoelasticity: Bone Biomechanics Lecture Slides). Instructors may also choose to assign for reading a Scientific American article by Angus Chen on bone nanostructure (12) as a foray into understanding structure-function relationships in biological materials, and also as a strong exemplar of journalistic scientific writing.
LECTURE
Previous lecture material
Immediately prior to the lesson on viscoelasticity of bone, students were exposed to lecture material covering the basics of bone biomechanics (Supporting Material S3. Bone Viscoelasticity: Bone Biomechanics Lecture Slides). The discussion associated with the slides included an emphasis on how analysis and comparison of stress-strain curves can provide useful information about biomechanical properties of bone. In this particular course, students had just learned about the properties of hysteresis and anisotropy--recent-historical and directional dependence, respectively, of the features of the stress-strain curve--in bone. Hysteresis in bone can be observed by differences in how it responds to stress depending on its most recent state; that is, for example, whether the bone had been previously heavily or lightly stressed. Anisotropy is evident in the fact that a force insufficient to break a long bone if applied along its axis may break the bone if applied perpendicularly: a difference in behavior of the bone depending upon the angle of the stress applied. For more in-depth discussion of these properties, see the accompanying Science Behind the Lesson article: "A Short Bone Biomechanics Primer: Background for a Lesson on Bone Viscoelasticity".
Viscoelasticity lecture scheme with suggested blocking and major questions.
Context:
For the duration of this scripted portion of the lesson, an image of a family of stress-strain curves depicting the viscoelastic behavior of bone is on display in front of the class on a single PowerPoint™ slide (Supporting Material S3. Bone Viscoelasticity: Bone Biomechanics Lecture Slides, slide 13). Frequent reference to this slide is made throughout the following.
Set-up:
The brief set-up to the lesson involves (re-)introducing students to Silly Putty®, a play material with which they are most likely already familiar. For this set-up, as a demonstration to the students, the instructor can alternately: a) slowly either stretch or crush the Silly Putty® at low strain rates (relatively small, low-rate force application) to result in large deformations: elongation to a point of stringiness and eventual breakage in the case of stretching; and flattening into a thin patty shape if fully crushing, and b): also bounce the Silly Putty® hard off of the desktop and wall to produce a very high strain rate, which results in little to no deformation, but rather a dramatic rebound. The slow-rate strain application--particularly if one lets the Silly Putty® partially "hang" off of a desk to allow it to creep--illustrates the viscous nature of the material; the high-rate strain application that the Silly Putty® experiences during bouncing illustrates its elasticity. (The existence of both properties in a single material is what makes it viscoelastic.)
Between the demonstrations of, first, the slow stretch and/or crush and, second, the bouncing, it can be fun to ask students what should happen to putty when a very high strain rate is applied by bouncing or hitting the putty very hard and quickly. The straightforward answer is that the putty should fail--that is, it should break, snap, or buckle under high rates of strain. But if they've played with Silly Putty® before, students will most likely answer that it will not. (And they are right.) This brief question-and-answer exchange can serve to highlight the importance both of hypothesizing/extrapolating and making actual experimental observations to confirm hypotheses, as part of the scientific process. Comparing the behavior of the Silly Putty® to other materials, such as glass, or ceramic (see Supporting Material S1. Bone Viscoelasticity: Bone Biomechanics Primer), or clay--which would collide inelastically with the desk to deform, and would not rebound--can underscore this point. (While clay can be readily used as a comparison material, we do not recommend performing a live-action demonstration with glass or ceramics.)
During this set-up, it is essential to continually quiz students orally as a group as to how the various manipulations of the Silly Putty® relate to bone biomechanics. Of great importance is reviewing the concepts of the plastic and elastic regions of the stress-strain curve. In the plastic region of the curve, Silly Putty® that has been slowly crushed or stretched will display its ductility. The elastic region of the curve is revealed and illustrated when the Silly Putty® is bounced. Asking students to identify which manipulation corresponds to which region of the curve serves to reinforce these concepts during the demonstration.
Discussion of experimental results:
Following the demonstration, the instructor can now make reference to the graph displaying a set, or "family," of stress-strain curves in response to different strain rates (Figure 2 and slide 13 of Supporting Material S3. Bone Viscoelasticity: Bone Biomechanics Lecture Slides). On the x-axis, the graph shows strain as a percentage of total length: a measure of the magnitude of deformation. On the y-axis is stress: a measure of load, or of applied force. Students may also consider this axis as showing the measured force that the bone itself exerts in response to a given applied strain-inducing force. All curves in the family exhibit a straight, linear elastic region, followed by a shallower-sloped plastic region after the yield point: the point at which the curve changes slope because the bone "yields" to the stress and deforms much more readily with continued load application. (Note that higher yield points are indicative of higher material strength; for more information, please see the accompanying Science Behind the Lesson article: "A Short Bone Biomechanics Primer: Background for a Lesson on Bone Viscoelasticity".)
It is important to note that the data are derived from an actual set of experiments by J.H. McElhaney on samples of cortical bone, where the investigator used a constant-velocity compression machine to apply strain to those samples at different rates, reported as percent deformation per second (3). McElhaney worked with strain rates ranging from 0.001% per second to 1500% per second. The resulting measured stress (i.e., the force) on the bone, is plotted in megapascals (MPa). Consequently, a family (or set) of stress-strain curves--one for each strain rate--is obtained.
It bears noting that this graph could be considered from a reversed perspective, with the understanding that to get a certain degree of strain, an investigator must apply a stress to the bone. Thus, an observer could also think of an experiment such as this one being a case of applying different rates of stress--that is, force or load--and measuring the strain, or deformation, that results. Either way, one would obtain a set of different stress-strain curves from different rates of loading, as is plotted in McElhaney's study (3).
At this point, the instructor can direct students to engage in a series of think-pair-share activities as a review of the bone biomechanics concepts previously discussed (Supporting Material S3. Bone Viscoelasticity: Bone Biomechanics Lecture Slides, slide 13). The first suggested question is: "At what loading rate does bone exhibit the greatest toughness?" As students consider the question, it can be helpful to remind them that toughness is a measure of energy absorption (1), which can be visualized as the area under the curve (2). Students should see that the greatest area under the curve, corresponding to the greatest toughness, comes at a strain rate of around 1% per second. Students may see--or if not, the instructor may point out--that many strain rates lower than 1% per second result in measurements of toughness that are similar to one another. It is important to note at this point that these strain rates would correspond to rates one might experience during everyday activities such as walking or running. For example, humans experience bone strain rates around 0.7% per second while walking, which become as high as 3.4% per second while sprinting (13). For quadrupeds, the numbers range from around 0.1% to 0.3%, respectively (14).
With this point regarding rate-dependent changes in bone properties in mind, students can then examine other biomechanical properties of bone at these low-to-moderate strain rates. Questions directed at points pursuant to other properties are embedded in the lecture slides (Supporting Material S3. Bone Viscoelasticity: Bone Biomechanics Lecture Slides, slide 13): "Under what conditions is bone strongest?"; "Under what conditions is bone most elastic?" Students should be able to see that for strain rates around 1% per second, bone is rather strong (i.e., has a relatively high yield point), somewhat stiff and elastic ("springy"), rather plastic (deformable), and features a high-strain failure point (breakage point) at the end of the curve.
Once students have listed these features, the instructor may then ask students to interpret these data. Students should be able to see that these properties, taken all together, under these moderate strain rates (or moderate loading rates) mean that the bone is less likely to fail. That is: the bone may bend, but not break.
After students have considered these questions, the instructor can then return to the question of what bone and Silly Putty® have in common. Silly Putty® and bone both feature a property called viscoelasticity, which is a dependence on rate of strain application in generating stress responses (see the accompanying Science Behind the Lesson article: "A Short Bone Biomechanics Primer: Background for a Lesson on Bone Viscoelasticity")--a "rate-dependent" property of a material. This property can be compared and contrasted with the already-discussed properties of hysteresis, a recent-history dependence of the behavior of a material, and anisotropy, a directional dependence of behavior. The concept of viscoelasticity can be presented simply as a response that depends on how fast a strain-inducing stress is applied--with specific response properties varying with the speed of applied loading.
The instructor can further emphasize the viscoelastic nature of bone by highlighting and comparing the shapes of the curves at the most extreme strain rates. First considering the lowest rate of loading, at 0.001% per second, the instructor can ask the students what properties the bone has when it is subjected to a very low-grade force (slow rate of strain) over a long period of time. Students should see that the bone is more flexible, and also yields at a low stress levels. Students in the class where this lesson was taught had previously seen the example of a skull belonging to a member of a Pre-Incan South American tribe, whose people who elongated their skull bones by binding infants' heads to a rigid board (please see, for reference, slide 11 of Supporting Material S3. Bone Viscoelasticity: Bone Biomechanics Lecture Slides); this deliberate deformation of skulls into a cone-like shape is thought to have served as a marker of in-group ethnic identity (see References 15 and 16). In that case, the plastic region of the stress-strain curve is achieved quite readily, leading to permanent deformation of the bone.
On the other hand, with the extremely high rates of loading, 300% or 1500% per second, the curves look quite opposite to what was observed at low rates of loading. (It is valuable to ask students whether these strain rates can be applied over a whole second; students should recognize that it would be impossible, as the bone would have been completely compressed well before the full second would have elapsed.) Students can once again be asked to list or call out the biomechanical properties of these high-strain-rate stress-strain curves. Students should be able to respond that these curves reveal steep slopes in their elastic regions (meaning greater bone stiffness), and much higher yield points (meaning higher bone strength). However, they should also see that there is a smaller area under the curve at these rates of strain (meaning that the bone is less tough), and that the post-yield-point plastic regions are much shorter (meaning that the bone will more readily fail).
To wrap up the discussion, students can be asked to engage in a final one-minute essay activity (slide 15 of Supporting Material S3. Bone Viscoelasticity: Bone Biomechanics Lecture Slides) where they consider real-world implications of viscoelasticity and anisotropy in the light of their interpretations of the related stress-strain curves. Several bone fracture patterns are displayed; students can consider how anisotropy and viscoelasticity apply to these patterns. Students should be able to recognize that higher strain rates might result from a jump or fall or other high-impact trauma (for example, see Reference 17, wherein an estimate of a strain rate of roughly 2500% per second during bone compression in a car accident is made). Students should be able further to see that the increased stiffness and strength found in bone at high strain rates--for a hard fall, for example--should in principle result in less overall bone deformation (cf. Silly Putty® bouncing); but that if the impact (force over short time) is great and sustained enough to induce a large strain at a high rate--during an automobile accident, for example--the bone will most likely fail by buckling or breaking. Much as Silly Putty® might if, rather than thrown to bounce off of a wall, it were hit hard with a hammer. Finally, students can see that applying forces from different angles can lead to different fracture patterns, and they may be able to make connections between the concept of anisotropy and this group of fracture patterns.
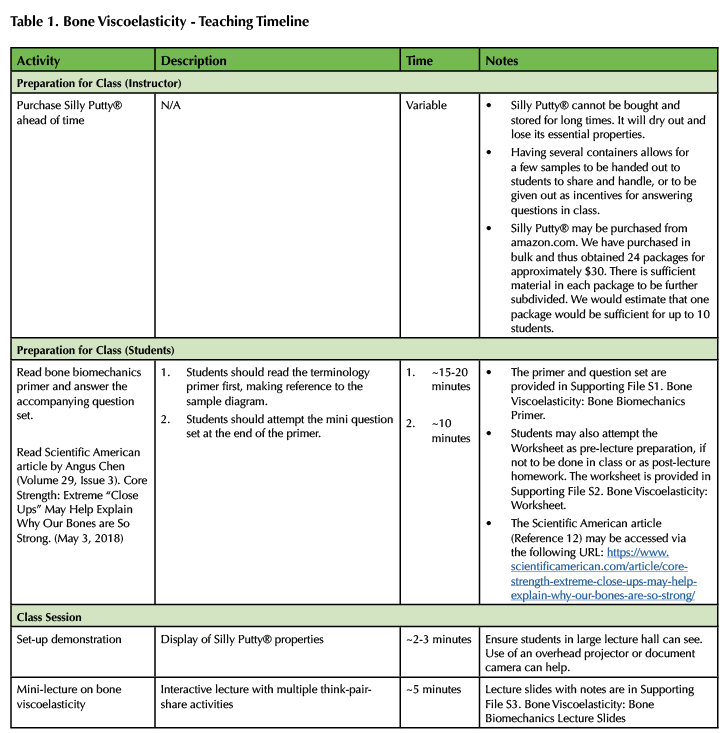
Table 1 Bone Viscoelasticity Teaching Timeline
TEACHING DISCUSSION
Lesson effectiveness
In the moment, the lesson appeared to capture student attention and to engage students intellectually in the material. There was a noticeable increase in student attention levels during the demonstration, and students asked many follow-up questions about the lesson, both during lecture time and during office hours. (Questions included: curiosity about which molecular components of bone render it viscoelastic; other ways of classifying bone's biomechanical properties; what other sources of stress might act on bone elastically and plastically; and how bone might recover from being in the plastic region.) Qualitative assessments of the effectiveness of this lesson in engaging students more directly in the material came from student comments. Many students found the lesson to be creative and interactive and thought that the demonstration made the learning of bone biomechanics more enjoyable and engaging. One student commenter called it "one of the most iconic demonstrations in this class." Another student summed up what we hoped would be the impression of the lesson, that the: "idea of using something so simple...to demonstrate such an amazing and complex property of the human body is quite clever and inventive."
Many students appreciated the visual aspect of the demonstration. The few students who did enjoy the opportunity to handle the Silly Putty® also commented positively on the tactile aspect of the experience. Unfortunately, because of the large enrollment of our course, distribution of a sample of Silly Putty® to each student is not practical in the lecture setting. However, distribution of samples for handling could work in our smaller-enrollment--14 students per section--lab sections (see below).
Possible improvements or adaptations
Lecture settings:
In smaller classrooms--or, as noted, in small-sized lab sections--it may be possible to allow students to work in groups, handling the Silly Putty® themselves. If the activity is done in groups, we recommend group sizes of four or five students to promote high interactivity. While in smaller classes it may be advantageous and readily achievable to pair higher- and lower-performing students, in our large course we are able to randomly assign students to groups ahead of class meeting times using the "Group" function within our learning management system (LMS). Using such a system, students could then be instructed to sit with their group as they enter the classroom.
In a small classroom, or in a laboratory environment, the lesson could also be more investigative, for example: having students make predictions about how the Silly Putty® shape and behavior would change with increasing force or speed of force application, then following the discussion up with a demonstration and student experimentation with the material.
In line with the fact that materials other than bone are mentioned for comparison in the primer (Supporting Material S1. Bone Viscoelasticity: Bone Biomechanics Primer), during the lesson (Supporting Material S3. Bone Viscoelasticity: Bone Biomechanics Lecture Slides), and in the question set (Supporting Material S4. Bone Viscoelasticity: Bone Biomechanics Assessment Questions), instructors could substitute rubber bands, paper clips, paper, and other low-cost materials for Silly Putty® and have students attempt to draw their own stress-strain curves based upon their empirical observations of relative force and displacement (deformation) in these materials. Since all of the suggested materials are common and inexpensive, they would be readily available to students outside of class for use in a homework assignment following the suggestions above for a small-classroom setting.
In a larger class, it is recommended to run the activity as a demonstration with Silly Putty®, as depicted in the video recording (Supporting Material S5. Bone Viscoelasticity: Bone Viscoelasticity Lecture Recording), and it may be possible to demonstrate the mechanical behavior of other materials as well, as time permits. Reports of demonstrations involving different varieties of commercially-available rubber balls (made of neoprene and polynorbornene), and even polyethylene bags and earplugs to illustrate both viscoelasticity and the properties of time-dependent creep and relaxation (18) are promising, but could require more preparation, equipment, and in-class time. Prof. Ryan Sochol's Bioinspired Advanced Manufacturing (BAM) laboratory at the University of Maryland has produced a very useful video (https://www.youtube.com/watch?v=5ZlH9pidAdc) that briefly mentions and illustrates the viscoelastic properties of Silly Putty®, and does discuss some biological applications, but does not relate these properties directly to bone (19). Nonetheless, these other resources could serve as useful and instructive supplements to the main lesson.
It would also be possible to consider, as a class, the possibility of bone's anisotropic properties applied to the viscoelasticity curves: that is, to ask students to predict how the family of curves depicted for the viscoelasticity discussion would look under different loading angles. This extension of the lesson would provide a nice dovetailing of two different properties (anisotropy and viscoelasticity) in the same stroke.
Laboratory settings:
It would be possible to further examine the concept of stress-strain curves with springs and force transducers, after the fashion of a typical physics-laboratory exercise exploring Hooke's law. Using yardsticks and force transducers, linear force-displacement curves (following Hooke's Law: F = kx) could be easily obtained with readily-available lab equipment. These portions of the experimentally-obtained curves would model the elastic regions of bone stress-strain curves for tensile (stretching) forces. With inexpensive, small, or expendable springs, students could extend the springs beyond their yield points for an exploration of the plastic regions of stress-strain curves, which is not typically done in Hooke's Law investigations. The resulting curves could then be used to review and reinforce the concepts relevant to understanding the elastic and plastic components of biomechanical stress-strain curves.
For courses in biomechanical-engineering and materials-sciences departments with the appropriate equipment and resources, it could be possible to design a laboratory exercise specifically around the idea of viscoelasticity using animal bones as the model and a servo-hydraulic materials-testing machine (see Reference 17) to carry out the strain application and stress measurements. Students could attempt to replicate the experiments and data of McElhaney (3), or, if sufficient variety of bone sources were available, students could be tasked with formulating hypotheses about the viscoelastic (or anisotropic) properties of bones from different species and then testing those hypotheses in the lab.
Finally, with high-speed videography, large-bore calipers, and pressure-sensing force transducers, it would also be possible to measure some aspects of the behavior of Silly Putty® as demonstrated in lecture. For example, students could video-record the bouncing of a ball of Silly Putty® against a force transducer. Using the recording, students could then determine the strain rate from the measured time of compression and accompanying image analysis of the balled-up Silly Putty®'s elastic deformation (or, the actual before-and-after caliper measurement of the Silly Putty® ball, in the case of plastic deformation) to plot strain against measured force at various calculated strain rates. Students could then plot the data and analyze the relationship between strain rate and yield strength, for example. With sufficient time to develop the lessons in detail, and a few resources at hand, the possibilities for extending this lesson into the laboratory abound.
Summary
Using the commonplace play material Silly Putty® as an (unexpected) model, we present here a short and simple demonstration of an interesting and unusual property of bone--viscoelasticity--that students might never before have encountered or considered. Nonetheless, the property of viscoelasticity has very real and impactful everyday applications to which students can readily make connections. We find it to be a very low-investment, but high-yield "iconic" demonstration that can greatly enliven a discussion of the skeletal system and its functions.
ACKNOWLEDGMENTS
We thank, from the University of Connecticut's Communications Office, Kristin Cole for organization of filming schedule and personnel and Angelina Reyes and Bret Eckhardt for videography, audio recording, and editing. We thank Ann Hamlin of the University of Connecticut's Department of Physiology and Neurobiology for providing a model bone for display. We thank Dr. Xinnian Chen for helpful comments on the manuscript. We thank Profs. Joseph LoTurco and Andrew Moiseff for support, and the students of PNB 2264/65 and PNB 2274/75, past and present, for their attention and efforts.
Silly Putty® is a registered trademark of Crayola Properties, Inc.:
Silly Putty®. 2012. Crayola LLC, Forks Township, Northampton County, Pennsylvania, USA.
PowerPoint™ is a trademark of Microsoft Redmond, WA.
SUPPORTING MATERIALS
- S1. Bone Viscoelasticity: Bone Biomechanics Primer
- S2: Bone Viscoelasticity: Bone Biomechanics Worksheet and Answer Key
- S3. Bone Viscoelasticity: Bone Biomechanics Lecture Slides
- S4. Bone Viscoelasticity: Bone Biomechanics Assessment Questions and Answer Key
- S5. Bone Viscoelasticity: Bone Biomechanics Lecture Recording
References
- Young, T. 1845. A Course of Lectures on Natural Philosophy and the Mechanical Arts. London: Taylor and Walton.
- Evans, F.G. 1955. Studies in Human Biomechanics. Ann. N.Y. Acad. Sci. 63(4):586-615.
- McElhaney, J.H. 1966. Dynamic response of bone and muscle tissue. J. Appl. Physiol. 21(4): 1231-1236. DOI: 10.1152/jappl.1966.21.4.1231
- Gardner, H. 1983. Frames of mind: The theory of multiple intelligences. New York: Basic Books.
- Kolb, D.A. 1984. Experiential Learning. Englewood Cliffs, NJ: Prentice-Hall
- Honey, P., Mumford, A. 1982. The Manual of Learning Styles. London: P. Honey.
- Rizzolatti, G., Craighero, L. 2004. The mirror-neuron system. Ann. Rev. Neurosci. 27(1): 169-192. DOI: 10.1146/annurev.neuro.27.070203.144230
- Newton, P.M., Miah, M. 2017. Evidence-Based Higher Education--Is the Learning styles 'Myth' Important?. Front. Psychol. 8: 444. DOI: 10.3389/fpsyg.2017.00444
- Husmann, P.R., O'Loughlin, V.D. 2018. Another nail in the coffin for learning styles? Disparities among undergraduate anatomy students' study strategies, class performance, and reported VARK learning styles. Anat. Sci. Educ. Mar 13 (epub ahead of print).
- DOI: 10.1002/ase.1777
- Krathwohl, D.R., Bloom, B.S., Masia, B.B. 1956. Taxonomy of Educational Objectives: The Classification of Educational Goals, Handbook II: Affective Domain. New York: David McKay Company, Inc.
- Hurtado, S., Han, J.C., Saenz, V.B., Espinosa, L.L., Cabrera, N.L., Cerna, O.S. 2007. Predicting transition and adjustment to college: Biomedical and behavioral science aspirants' and minority students' first year of college. Res. Higher Ed. 48(7):841-887.
- Chen, A. "Core Strength: Extreme "Close Ups" May Help Explain Why Our Bones Are So Strong" Scientific American. 3 May 2018. Web. Accessed 10 June 2018
- Burr, D.B., Milgrom, I.C., Fyhrie, D., Forwood, M., Nyska, M., Finestone, A., Hoshaw, S., Saiag, E., Simkin, A. 1996. In Vivo measurement of Human Tibial Strains during Vigorous Activity. Bone 18(5):405-410.
- Rubin, C.T., Lanyon, L.E. 1982. Limb Mechanics as a Function of Speed and Gait: A Study of Functional Strains in the Radius and Tibia of a Horse and Dog. J. Exp. Biol. 101:187-211.
- Torres-Rouff, C. 2002. Cranial Vault Modification and Ethnicity in Middle Horizon San Pedro de Atacama, Chile. Curr. Anthropol. 43(1):163-171.
- Tubbs, R.S., Salter, E.G., Oakes, W.J. 2006. Artificial deformation of the human skull: a review. Clin. Anat. 19(4):372-377. DOI: 10.1002/ca.20177
- Hansen, U., Zioupos, P., Simpson, R., Currey, J.D, Hynd, D. 2008. The Effect of Strain Rate on the Mechanical Properties of Human Cortical Bone. J. Biomech. Eng. 130(1):011011-1-5.
- DOI: 10.1115/1.2838032
- Mano, J. 2006. Classroom Methods for Demonstrating, Modelling, and Analysing the Viscoelasticity of Solids. Int. J. Engng. Ed. 22(5):970-978.
- BAM lab. 2013, Sep. 18. Introduction to Viscoelasticity. Retrieved from https://www.youtube.com/watch?v=5ZlH9pidAdc Web. Accessed 20 March 2018.
Article Files
Login to access supporting documents
What do Bone and Silly Putty® have in Common?: A Lesson on Bone Viscoelasticity(PDF | 256 KB)
S1.BoneViscoelasticity-BoneBiomechanicsPrimer_0.docx(DOCX | 469 KB)
S2.BoneViscoelasticity-BoneBiomechanicsWorksheet_AnswerKey_0.docx(DOCX | 20 KB)
S3.BoneViscoelasticity-BoneBioMechanicsLectureSlides_0.pptx(PPTX | 20 MB)
S4.BoneViscoelasticity-BoneBiomechanicsAssessmentQuestions_withKEY_0.docx(DOCX | 594 KB)
S5.BoneViscoelasticity-LectureRecording.mp4(MP4 | 88 MB)
- License terms
Comments
Comments
There are no comments on this resource.