Breaking Bricks: A Hands-On Model of Enzyme Kinetics and Inhibition
Editor: Leocadia Paliulis
Published online:
Abstract
Enzyme kinetics and the impacts of inhibitors on the enzyme's maximal velocity and ability to bind substrates are important topics in cell biology and biochemistry. However, these topics can be difficult for students to grasp when instructed using a traditional lecture format. Teaching biological concepts using physical models has been shown to improve to student comprehension and engagement with the topic. We have developed a pre-lab activity that uses plastic building bricks and student "enzymes" to expose students to these concepts prior to conducting enzyme assays at the bench. Small groups of students take turns acting as an enzyme that catalyzes a hydrolysis reaction with increasing substrate concentration in the presence and absence of a competitive inhibitor. Students graph brick breaking rate data and make observations about the effect of changing parameters on key metrics. We conclude the activity with a class discussion on their observations. According to survey data, our students show an increase in the ability to answer conceptual and graphical questions correctly after completing the activity and corresponding material. Moreover, the majority of students thought that the activity was moderately or greatly helpful at increasing their understanding of key concepts. This kinesthetic active learning approach provides an engaging and fun way to introduce students to modeling enzyme kinetics and is adaptable to any class or laboratory setting.
Primary image: Breaking Bricks: A Hands-on Model of Enzyme Kinetics and Inhibition. Enzyme-catalyzed hydrolysis of a disaccharide into two monosaccharides is modeled by 2x2 plastic building bricks, with students’ hands representing the enzyme.
Citation
Darling LEO, Goss JW, Roden JA. 2021. Breaking bricks: A hands-on model of enzyme kinetics and inhibition. CourseSource. https://doi.org/10.24918/cs.2021.16
Society Learning Goals
Biochemistry and Molecular Biology
- Energy is required and transformed in biological systems
- How do enzymes catalyze biological reactions?
- Macromolecular Structure Determines Function and Regulation
- How is the biological activity of macromolecules regulated?
Cell Biology
- Matter & Energy Transformation
- How do cells transform energy and cycle matter?
Lesson Learning Goals
From Biochemistry and Molecular Biology Learning Framework:
- "How do enzymes catalyze biological reactions?"
- "How is the biological activity of macromolecules regulated?"
From Cell Biology Learning Framework:
- "How do cells transform energy and cycle matter?"
From Science Process Learning Skills Framework:
- Predicting outcomes
- Interpreting results/data
- Analyzing data
- Displaying/modeling results/data
- Gathering data/making observations
- Formulating hypotheses
Lesson Learning Objectives
Students will be able to:
- Describe the effect of increasing substrate concentration on the velocity of an enzyme-catalyzed reaction.
- Define and estimate the maximum velocity of the reaction (Vmax) and the Michaelis-Menten constant (Km) for a given enzyme.
- Predict the effect of a noncompetitive inhibitor and a competitive inhibitor on the Vmax and the Km for a given enzyme.
Article Context
Course
Article Type
Course Level
Bloom's Cognitive Level
Vision and Change Core Competencies
Vision and Change Core Concepts
Class Type
Class Size
Audience
Lesson Length
Pedagogical Approaches
Principles of How People Learn
Assessment Type
INTRODUCTION
Enzyme kinetics and inhibition are fundamental topics in cell biology and biochemistry. Many textbooks used for these courses include a discussion of the structure of enzymes and their active and allosteric sites, the effect of increasing substrate concentration on the rate of reaction, and the definitions of the theoretical maximal velocity of the reaction (Vmax) and the Michaelis constant (Km), the substrate concentration at which half the active sites of the enzyme are filled (1). The mathematical model of enzyme kinetics developed by Leonor Michaelis and Maud Menten and the Lineweaver-Burk (double reciprocal) plot enable estimation and calculation, respectively, of Vmax and Km for an enzyme-catalyzed reaction. In addition to their relevance for biochemistry, the analytical methods used to interpret simple enzyme kinetics data are more broadly relevant. The American Association for the Advancement of Science's Vision and Change report describes the ability to use quantitative reasoning and mathematical modeling on biological data as one of the core competencies that should be included in undergraduate biology education (2). However, many students struggle to understand these key concepts. A survey of undergraduate biochemistry students from several different institutions demonstrated that some respondents still held misconceptions about characteristics of substrate binding to the active site of an enzyme even after they had been taught the material (3). Additionally, through several semi-structured interviews, it was clear that students had differing levels of understanding of the action of inhibitors on an enzyme reaction scheme (4). Finally, students' ability to describe the effect of non-competitive and uncompetitive inhibitors was less nuanced than their descriptions of competitive inhibition (5). Thus, incorporating multiple methods to expose, engage, and guide students through these concepts and analyses would likely improve student understanding.
Many studies suggest that teaching with active learning, which includes both activities and group discussion as opposed to passive listening to an expert, improves student outcomes. A meta-analysis comparing student examination scores throughout science, mathematics, engineering, and technology courses demonstrates that student performance is higher in courses that incorporate active learning (6). In addition, students in an introductory biology course that included active learning activities reported significantly more learning, increased interest in the course material, and more stimulation in the classroom than a cohort taught by the same instructor in a lecture-based format (7). While active learning can take many forms, incorporating physical models of biological phenomena can have marked effects on student learning. For example, female undergraduates who spent a discussion section manipulating physical models showed significant improvement in understanding both by quiz score and by student assessment of their own learning (8). In addition, male and female students in a cell and molecular biology class showed significantly higher learning gains on concepts that were taught using model-based activities than concepts taught without models when tested using a Central Dogma Concept Inventory (9). Finally, over 80% of the students that used a strategy of Process-Oriented Guided Inquiry Learning (POGIL) to build knowledge in a biochemistry course thought that these POGIL activities were helpful to their learning and recommended them for use in future versions of the course (10). Thus, incorporating student-centered, constructive activities into biology and biochemistry learning has many benefits for students.
We formerly began our laboratory study of enzyme kinetics with short presentation-based lectures at the start of each lab session, which included examples and discussion questions for students. However, this strategy did not engage students, who remained confused about key measurements and how to graphically represent them until they collected and began to analyze their own experimental data. We decided to develop a more student-centered lesson that incorporated active learning. Several different physical representations and models of enzyme kinetics have been developed previously. In one activity, students create enzymes out of modeling clay and manipulate them to represent shape changes during reactions (11). Two activities simulate enzymatic transport by having students transfer marbles between containers (12) or remove beans from a bag (13). Finally, other activities ask students to add pennies into a tube from a mixture including other coins as "inhibitors" (14) or unscrew nuts from bolts (15) to model Michaelis-Menten kinetics. However, none of these hands-on models of enzyme kinetics directly model a hydrolysis reaction, and many are directed at non-major or chemistry major student populations.
In our cell biology course, the attached laboratory uses the enzyme beta-galactosidase as a model and examines the effects of inhibitors on enzyme kinetics. Beta-galactosidase is part of the Escherichia coli lac operon, a model of gene regulation first described by François Jacob and Jacques Monod (16). Beta-galactosidase catalyzes the cleavage of the disaccharide lactose into two monosaccharides, galactose and glucose, as well as a transgalactosylase reaction converting lactose to allolactose (17). The crystal structure of beta-galactosidase bound to several different inhibitors, including the competitive inhibitor isopropyl β-D-1-thiogalactopyranoside (IPTG), has been solved, and the Michaelis constant (Km) of the enzyme in the presence of these inhibitors has been measured (18). Over the course of several weeks, our students follow a series of protocols to purify beta-galactosidase from E. coli and spectroscopically measure the rate of enzyme-catalyzed cleavage of a substrate. They then select a potential inhibitor, predict its effect on the reaction, design an experiment, and observe and analyze their findings.
In this lesson, we describe a hands-on model that introduces key topics related to kinetics of a hydrolysis reaction similar to that catalyzed by beta-galactosidase, along with the effects of competitive and noncompetitive inhibitors on the reaction. We have created a physical model of this enzyme catalyzed reaction using students as enzymes and a familiar object as the substrate (plastic building bricks such as LEGOTM). As part of the lesson, we incorporated opportunities for groups of students to create simple graphs with this model data and thought questions to help students discuss the successes and limitations of this model.
Intended Audience
This lesson is appropriate for an intermediate undergraduate cell/molecular biology or biochemistry course that discusses the topic of enzyme kinetics, particularly in the context of an enzyme that catalyzes a hydrolysis reaction. The lesson has been successfully implemented in a 50-60 student intermediate Cell Biology course during the 12-person attached laboratory sections at a small, selective, women's liberal arts college. Our course primarily enrolls biological sciences, biochemistry, and neuroscience majors who are fulfilling a major requirement, although a small subset of non-major pre-health students enroll in the course due to interest. Students are required to have taken 2 semesters of college chemistry prior to enrolling in the course. According to our student survey data, the majority of the students have not yet taken or are currently co-enrolled in a biochemistry course, another course where enzyme kinetics is covered in our curriculum.
Required Learning Time
This lesson can be completed in an hour to 75 minutes as written, though modifications to add or eliminate testing and discussion of various potential inhibitors could shorten or extend this time. In our course, this lesson was spread over parts of two lab sessions. In the first 45-minute session, pairs or small groups of students used the hands-on model to collect and graph data in the presence and absence of inhibitors. After completing the activity and before the next lab session, each student independently answered thought questions. At the beginning of the next laboratory session (one week later), the instructor facilitated a discussion of the activity, what students learned, and extensions and drawbacks of the model for 20-30 minutes prior to beginning wet-bench enzyme kinetic experiments with a purified enzyme and varying substrate concentrations.
Prerequisite Student Knowledge
Prior to completing this lesson, students should familiarize themselves with the basic concepts of enzyme kinetics and inhibition, which are typically described in many cell biology and biochemistry textbooks, such as Molecular Biology of the Cell (1). In our course, relevant information about the Michaelis-Menten and Lineweaver-Burk plots, definitions of the Michaelis constant (Km) and maximum velocity (Vmax) of an enzyme-catalyzed reaction, the effects of inhibitors on these values are provided in the electronic lab manual. Students are asked to read the lab manual prior to the lab session where the lesson is taught. Key concepts in enzyme kinetics are also discussed in the lecture portion of this course at this point in the semester.
Additionally, students should be comfortable taking numerical data and creating a simple scatterplot and adding a trendline using a graphing program.
Prerequisite Teacher Knowledge
Instructors should have a good understanding of the key enzyme kinetics terms and concepts described for students above. In addition, they should be able to take numerical data (substrate concentration and product formation rate) and create a scatter plot and be able to troubleshoot student graphing issues.
SCIENTIFIC TEACHING THEMES
Active Learning
In this lesson, students use a hands-on model for enzyme kinetics as well as collecting and graphing their own data. This strategy encourages students to work together to simulate an enzyme-catalyzed reaction, analyze the data, and compare what they observe to what they have learned about the function and structure of real enzymes. Cooperative, constructivist-based activities that are open ended, require interpretation, and have answers that are not directly found in a textbook were proposed as strategies that are effective to help students build knowledge in biology (19). Moreover, active learning using physical models of a biological concept has been shown to improve student scores on questions that test those concepts to a greater degree than concepts where other types of active learning, such as group problem solving or clicker questions, are used (9). These types of activities can be included in Process-Oriented Guided Inquiry Learning (POGIL), in which small groups of students use active learning activities led by students and facilitated by the instructor to develop their own understanding, explore models, use discipline-specific knowledge, and develop important skills (20).
Assessment
Students in our course have multiple opportunities to demonstrate their understanding of enzyme kinetics and inhibition. During the activity, students are prompted to answer questions on the activity worksheet and discuss answers with their lab partners. At the end of the activity, students complete wrap-up questions, which are discussed as a class. Prior to the next week, students answer pre-lab discussion questions focused on the concepts introduced in the lesson. In our course, after completing this activity students conduct an experiment using beta-galactosidase that they have purified from E. coli to catalyze the hydrolysis of a substrate in the presence and absence of a potential inhibitor. Laboratory assignments ask students to examine and graph the data and use these measurements to discuss whether a particular chemical is an inhibitor, and if so, what type. We also assess student learning of these concepts on summative exams and problem sets in the lecture portion of the course.
In designing the Breaking Bricks activity and its corresponding assessments, we focused on addressing two core concepts from Vision and Change (2). First, our activity and corresponding laboratory experiments expose students to the idea that protein structure defines its function. Second, we connect the biological process of enzyme-catalyzed hydrolysis into a larger framework of chemical transformation pathways that are governed by the laws of thermodynamics. In addition, our activity allows our intermediate-level students to practice Vision and Change core competencies, such as applying quantitative analysis and mathematical reasoning to biological data, ability to use models to explain complex systems, and to communicate scientific information (2).
We evaluated whether this activity, in combination with other material discussed in the lecture portion of this course, was able to help students learn these concepts. We administered an anonymous survey to our most recent cohort of 37 students prior to the start of the laboratory period to evaluate students' baseline knowledge of enzyme kinetics and inhibition. One week after the lesson, we asked the same questions again. In the second survey, we also asked several attitudinal questions about the activity, modeled after questions used in a previously published assessment of an enzyme kinetics activity (12). The survey protocol was reviewed and was determined to be exempt from human subjects protections review by the Wellesley College IRB, under §46.101 b, Exemption 1.
Inclusive Teaching
This activity uses physical models and student participation, and instructors encourage all students to act out the part of enzymes. In addition, using familiar objects (plastic building bricks) as the substrates and a human action in place of the enzyme may make the concept of enzyme kinetics more relatable and approachable. This simulation is performed in small groups, where students can play several roles in conducting the simulation (including timekeeper, data recorder, student "enzyme", or observer). Therefore, this activity could be modified to allow differently-abled students to participate depending on their needs by having the instructor and/or student group members discuss how all students can be involved prior to beginning the simulation. For example, in one of our classes, we had a visually impaired student who enjoyed being the "enzyme" because this kinesthetic activity doesn't require seeing the bricks.
This activity also provides multiple ways for students to answer questions about the material. When students complete the activity, they first answer questions in writing independently or in small groups, and then questions are discussed orally as a larger group. This technique allows all students to have the opportunity to participate. Structured discussion of individual student responses to the post-activity conceptual questions also allows students to teach each other.
LESSON PLAN
This lesson serves as a hands-on, conceptual model of enzyme kinetics in the presence and absence of an inhibitor. In the hands-on activity, students first define the enzyme, the substrate, and the products, then run the reaction without an inhibitor, create a simple graph of the results, and repeat the experiment again in the presence of the inhibitor. We conclude with a whole class discussion about the results of the activity, its benefits and limitations, and its connections to the experiments we will do in the lab.
Pre-Class preparation
Instructor preparation
Prior to the lesson, instructors should assemble the substrate building bricks into pairs, and sort them into containers holding about 60 substrates per container. We used 2 different colors for the substrates to model the glucose and galactose monosaccharides that make up the disaccharide lactose (Figure 1). Instructors should glue together enough pairs of building bricks in a different, third color than the substrate (see Figure 1) to provide 15 inhibitors to each group of students. Through multiple trials, we determined that 15 inhibitor molecules added to each reaction approximates the human "enzyme's" Ki (inhibitor constant, an inhibitor concentration where, under saturating substrate concentrations, half-maximal inhibition of the enzyme is reached). Creating inhibitor brick pairs in a different color of bricks than the substrate does not affect the outcome of the activity, but significantly shortens the cleanup and reassembly time required. Finally, instructors should make enough copies of the activity handout (Supporting File S1. Breaking Bricks – Student Activity Handout) for each student.

Figure 1. Examples of physical objects used in this activity. (A) Drawstring bag, approximately 6”x10”, (B) 2x2 plastic building bricks in two colors stacked together to represent disaccharide substrates, and (C) 2x2 plastic building bricks glued together to represent inhibitors.
Student preparation
In advance of the lesson, students should read background information on enzyme kinetics, including effects of substrate concentration, definitions of Km and Vmax, and the effect of competitive and non-competitive inhibition on these reactions. In our course, we provided this information to students in our online laboratory manual, but it could also be disseminated as a textbook reading or a video mini-lecture depending on the course's needs and structure. Optionally, instructors can ask students to anonymously complete a pre-activity assessment (an example is provided as Supporting File S5. Breaking Bricks – Pre-Assessment) to measure the level of understanding of key concepts in enzyme kinetics prior to completing the activity.
In-Class Activities
Introducing and defining the system (5 minutes)
The instructor begins this lesson by describing the setup and key concepts of the model (Supporting File S2. Breaking Bricks – Introductory Slides for Enzyme Kinetics Activity). Small groups of 2-3 students define the enzyme, substrate, product, and concept of saturation in this model of an enzyme-catalyzed reaction. These groups then take a container full of substrate to their workspace. Ideally, the classroom or laboratory space in which the lesson is taught should have enough surface area for each group of students to place, count, and sort the products and excess substrate, so lab benches or larger desks and tables are better for this activity than tablet chairs.
Enzyme kinetics in the absence of an inhibitor (10-15 minutes)
The instructor should begin the activity with some instructions and ground rules for how to conduct the model experiment. We typically discuss the importance of keeping the motions of the "enzyme" consistent across volunteers, such as whether the student "enzyme's" eyes should be opened or closed, whether they should separate the bricks inside the container, and whether the products should be added back to the container. Each round of the activity starts with a designated number of substrate molecules (stacked pairs of plastic 2x2 bricks) in the container, mimicking different substrate concentrations. In our experience, trials with 5, 12, 20, 40, and 60 substrate molecules work well for five rounds total. In each round, one student will act as an "enzyme" to reach into the container and separate as many pairs of bricks into single bricks as they can in 30 seconds. The other member(s) of the group act(s) as the timekeeper. After the time is up, the group should count and record how many substrate molecules (pairs of bricks) were separated for that particular concentration. If the student "enzyme" separates all of the substrates in the container before the time is up, they should record the number of substrates with which they started. Instructors should also inform the group that if multiple students act as the "enzyme", it is important for each person to record their own data for each substrate concentration (each round), as each "enzyme" has their own Vmax. Students can refer to the handout (Supporting File S1. Breaking Bricks – Student Activity Handout) while they are completing this activity.
While students are performing the activity to separate bricks, the instructor can circulate through groups of students, observing how they are doing with the activity and asking about any questions that have come up. After all substrate concentrations have been tested, instructors should ask students to create a graphical representation of their data with a scatterplot in the form of a Michaelis-Menten plot, graphing substrate concentration on the x-axis and reaction rate (paired bricks separated per second) on the y-axis. This graph can be created either with a hand-drawn sketch or by inputting the data into a spreadsheet/graphing program (Supporting File S3. Breaking Bricks – Enzyme Kinetics Activity Datasheet).
Enzyme kinetics in the presence of a competitive inhibitor (10-15 minutes)
Once students have completed the first part of the activity, created a graph, and answered the corresponding thought questions, the instructor should pause the activity. At this point, the instructor should ask students, either in groups or as a class, about their observations on the effects of increased substrate on enzyme rate. Instructors should highlight and reinforce students' responses that demonstrate understanding that at low substrate concentrations, an increase in substrate leads to an increase in reaction rate, but at high substrate concentration, the reaction rate remains essentially the same. To transition to the next part of the activity, the instructor should then lead a brief discussion on competitive inhibition and its effect on an enzyme catalyzed reaction. One strategy we have used is to ask students what they might use as a competitive inhibitor or whether students think it is reasonable to use glued-together bricks as a representation for a competitive inhibitor.
The instructor will then distribute fifteen inhibitor brick pairs to each group of students. Students will repeat the enzyme cleavage simulation using the same five substrate concentrations, 30-second reaction time, and keep consistent the other motions of the reaction as described above but will add the fifteen inhibitor molecules to the container along with the substrate molecules. Each round will thus have a different substrate concentration (5, 12, 20, 40, or 60 brick pairs) but a fixed inhibitor concentration (15 glued brick pairs). The instructor should remind students that they should keep their "enzyme" motions consistent with those from the uninhibited reaction. The instructor should provide two additional instructions for this part of the activity. If the inhibitor bricks are a different color or otherwise marked in some way, they should advise student "enzymes" to complete the reaction with their eyes closed. The instructor should also recommend that if the student "enzyme" is unable to pull apart the pair of bricks (whether it is an inhibitor molecule or just a stubbornly stuck substrate) that they place it back into the container.
At the conclusion of this portion of the simulation, students should then note their observations about how this simulation was similar or different to the reaction without inhibitor. Students should then graph the values as a new series on the same axes as the uninhibited enzyme (Supporting File S3. Breaking Bricks – Enzyme Kinetics Activity Datasheet). In small groups, they should examine these graphs and consider how the curves are similar to and different from each other, considering both the shape of the graphs and estimates of the Km and Vmax for each reaction. In group discussions, instructors can reinforce student observations describing a decrease in reaction rate of inhibited reactions at low substrate concentrations and minimal effect of the inhibitor on the reaction rate at high concentrations.
Non-competitive inhibition and other thought questions (5-10 minutes)
After completion of the two different enzyme activity simulations, instructors should provide student groups with time to consider how they might adapt this model to show the effect of a non-competitive inhibitor. In this type of inhibition, the enzyme is able to bind to the substrate with similar affinity but the ability to stabilize the transition state and catalyze the reaction is reduced. Some examples our students proposed that model non-competitive inhibition in the breaking brick simulation are to run the experiment wearing a laboratory glove, or taping several fingers together. Note that if class time allows, students can incorporate their strategy to model non-competitive inhibition by running enzyme brick simulation with their suggested modifications and identical substrate concentrations, but this will add 15-20 minutes to the total time of this activity. At the end of the activity, student groups should discuss extensions and caveats to using this model and note down any unanswered questions about enzyme kinetics (Supporting File S1. Breaking Bricks – Student Activity Handout).
To facilitate reuse of these materials by another group, students should reassemble the 60 substrate pairs and return them to their container. They should also sort out all of the inhibitor brick pairs used and return them to the instructor.
Wrap-Up and Assessment Activities
Concept-based questions (5-10 minutes, outside of class)
There are many options to assess whether students understand the concepts modeled in this activity, including the effect of adding additional substrate on the rate of the reaction and the effect of a competitive inhibitor on the enzyme. Instructors in our course provide students with several conceptual questions to answer individually prior to the next course meeting (Supporting File S4. Breaking Bricks – Discussion Questions). At the beginning of the second class period, small groups of students hold a structured discussion about these conceptual questions modified from the ORID focused conversation method (21). Briefly, each student takes a turn to independently share their answers, then each student shares a reflection on differences between answers, and then the group synthesizes final answers. Optionally, if the pre-activity survey described above is used prior to the activity, instructors could ask students to complete a post-activity survey (for an example see Supporting File S6. Breaking Bricks – Post-Assessment).
Conceptual observations and thought questions (15-20 minutes)
As a way to draw connections between this activity and the action of a real enzyme, and as a formative assessment, instructors should moderate a group discussion of the final set of questions on the handout (Supporting File S1. Breaking Bricks – Student Activity Handout). Student responses to questions about strengths and weaknesses of the model often include both the benefit of a hands-on model for a complex reaction, and the caveat that our student "enzymes" learn over the course of the simulation, which can change their rate. Asking students about unanswered questions on enzyme kinetics allows them to connect what they have learned from the classroom portion of our course or other experiences to the lab. This discussion also helps instructors identify whether any major misconceptions are still held by students. In our course, instructors lead this discussion in the lab section one week after the activity to allow students time to reflect on their answers. However, this larger discussion could also be done immediately after the small groups have an opportunity to come up with answers, ensuring that all students are able to engage with the questions even if they are unwilling to speak up in a large group.
List of Materials Needed to Complete this Lesson:
- 2x2 plastic building bricks (such as LEGOTM) in three different colors. At least 60 bricks of each main color and 30 bricks of inhibitor color are needed per group of students.
- Fast-acting adhesive to glue together inhibitor bricks.
- Small opaque bags or boxes to hold and store bricks. We used cloth drawstring bags (~6" by 10"), in which we also stored the materials between semesters.
- Printouts/digital copies of activity handout (Supporting File S1. Breaking Bricks – Student Activity Handout)
- Timers or stopwatches.
- Access to a computer and graphing software OR graph paper.
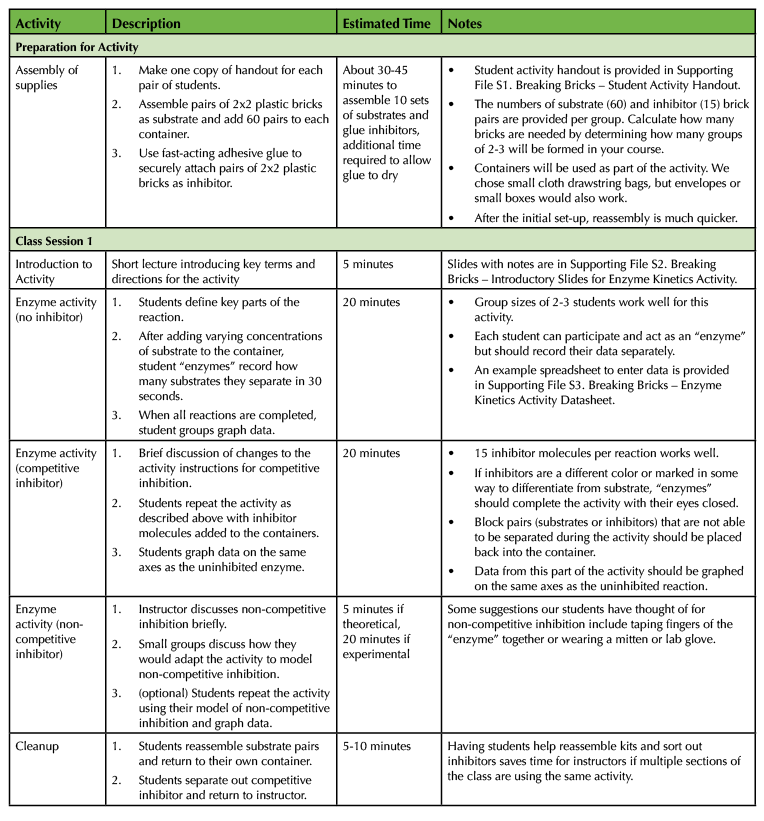
Table 1. Breaking Bricks lesson teaching timeline.

Table 1. Breaking Bricks lesson teaching timeline (continued).
TEACHING DISCUSSION
Our objective for this lesson was to create a more engaging, physical, and active introduction to key concepts in enzyme kinetics in the presence and absence of a competitive inhibitor. We believe that this lesson was effective at introducing students to key concepts in enzyme kinetics and inhibition by modeling a hydrolysis reaction.
Effectiveness of the Lesson
Comparisons between our pre-lesson and post-lesson survey responses suggest that, overall, students appear to better understand key concepts in enzyme kinetics after the lesson and corresponding instruction in the lecture portion of the course. In our post-lesson assessment, significantly more students were able to correctly answer questions about the effect of inhibitors and correctly interpret the Michaelis-Menten and Lineweaver-Burk plots (Figure 2). This increase in comprehension is likely due to a combination of material covered in the lecture portion of our course and accompanying problem set, as well as engaging in the Breaking Bricks activity in the lab. However, the proportion of students correctly answering questions about how to interpret the Lineweaver-Burk plot was somewhat lower than the corresponding Michaelis-Menten representation of the data even after the lesson (Figure 2). This is not surprising, since creating and analyzing Lineweaver-Burk plots was not included in the Breaking Bricks activity, though it was discussed in the lecture. We discuss how to create and manipulate these types of graphs when analyzing the beta-galactosidase experimental data students collect in the second laboratory session which follows after the activity. Graphing of enzyme kinetics data using the Lineweaver-Burk plot could be easily added to the data analysis portion of the "Breaking Bricks" activity if understanding and interpreting this type of graph is not addressed elsewhere in the course.

Figure 2. More students correctly answered conceptual and graphical questions about enzyme catalysis after the lesson. Anonymous surveys were completed by students electronically both as a (A) Pre-lesson assessment (n=36) before students completed the activity, and a (B) Post-lesson assessment (n=34), a week after the activity was conducted. The survey contained identical questions including two conceptual questions and four questions about the interpretation of Michaelis-Menten (M-M) and Lineweaver-Burk (LB) graphs. Distributions between pre-assessment and post-assessment were significantly different for all questions (Chi square, p=0.0034 for “Increasing substrate concentration” and p<0.0001 for all other questions).
In our post-activity survey, we incorporated a set of published attitudinal questions used to assess a related physical model of enzyme kinetics (12) to evaluate whether our students found the activity worthwhile. The majority of students perceived that this lesson was greatly or moderately helpful for visualization of several different key concepts of enzyme kinetics, including the effect of increasing substrate concentration on the rate of reaction, the concept of Vmax, and the effect of adding a competitive inhibitor to the reaction (Figure 3). We note that survey data showed that overall students found the lesson less helpful in visualizing Km. We expect that this is because the increase in Km observed in the presence of a competitive inhibitor is more easily visualized on a Lineweaver-Burk plot or with a broader array of substrate concentrations than used in this lesson.

Figure 3. Students found that the breaking bricks activity helped them visualize key concepts in enzyme kinetics. Several attitudinal questions were included on the anonymous post-lesson survey completed electronically by students a week after the lesson (n=34).
Student Reactions to the Lesson
Overall, students and faculty instructors have enjoyed completing this lesson throughout the three years we have used it. Enzyme kinetics can be a difficult topic for instructors to teach and students to understand, so using a physical model with familiar objects such as plastic bricks to model hydrolysis of a disaccharide made understanding the underlying concepts easier for our students. In fact, in an open-ended comment on our post-lesson survey, one student remarked: "This activity helped me conceptualize and start connecting kinetics concepts. Prior to this activity enzyme kinetics felt very abstract and thus very confusing."
In addition, many students found the physical act of disassembling the bricks enjoyable, especially since building with plastic blocks can be a common childhood pastime. We note that there was usually a large amount of laughter, shouting, and friendly competition for "enzymes" with the greatest Vmax from students (and instructors!) while engaged in the activity in our classes. One student said, "I think I didn't really understand how all the concepts linked until I created the graphs, which could have been done with any other data, but using the LEGOs was very interactive and fun!" As instructors, we also found ourselves in the final discussion talking with students about how humans acting as enzymes were not perfectly representative of how an enzyme works mechanistically, suggesting that students were engaged in higher order comparison and evaluation of this activity.
Suggestions for improvements or adaptations
While we have used this lesson as an introduction to enzyme kinetics in our course-based laboratory focused on beta-galactosidase, this lesson is broadly adaptable as a physical model of enzyme kinetics and inhibition for any cell biology or beginning biochemistry course in either a classroom or laboratory setting. We believe that this lesson works best when incorporated as a simple, physical introduction to the topic of enzyme kinetics prior to more detailed experiments or problem-based learning. While we have not taught this activity using the POGIL format as described by Moog et al, (20), our lesson could be easily reorganized such that students complete the "Exploration" phase of running the breaking brick simulation prior to "Term Introduction" of defining the enzyme, substrate, and product. Similar to the outcomes described in the POGIL format, students conducting this activity have the opportunity to practice hypothesis creation, data analysis, and scientific communication in addition to building content knowledge about enzyme kinetics.
Timing of when this activity is integrated into the curriculum is important for both student learning and student motivation. In a previous iteration of the course, we included this lesson after students had already conducted their beta-galactosidase experiment in the presence and absence of the competitive inhibitor IPTG and fit their experimental data to the Michaelis-Menten and Lineweaver-Burk plots. In that course structure, the majority of the students had already spent time thinking about enzyme kinetics and how inhibitors affect the enzyme and thus thought that the activity was too simplistic. Students in the revised version of the course appreciate the simplicity of the breaking brick activity as a beneficial and relatable introduction to enzyme kinetics before delving into a more complicated system like that of beta-galactosidase.
We have separated the act of doing the breaking brick activity and the wrap-up discussion into two sessions based on other experiments that are ongoing in our laboratory course. However, this lesson could be taught all in one 75-minute class period. In this case, we recommend that the instructor introduce the lesson, followed by the class dividing into small groups of students to complete the breaking brick activity, generate graphs, and think through their extensions, limitations and concerns. The class would end by reconvening as a group for a final discussion. In addition, if time permits, students could also test out their proposed ideas for non-competitive inhibition and graph them to see whether the data follows the model which predicts a change in Vmax and no change in Km.
While we typically use this activity in an in-person laboratory setting, we believe that the Breaking Bricks activity and its suggested extensions could also be helpful for a remote-taught course or replacement for an enzyme-kinetics laboratory activity. Materials like plastic building bricks may be available at home for some students or are easily ordered and shipped to them by their home institution. Students could perform the activity as described and record their data individually either synchronously or asynchronously. Student groups can then work together to pool data on shared spreadsheets, answer conceptual questions, and complete ORID-style structured discussions about their observations.
SUPPORTING MATERIALS
- S1. Breaking Bricks – Student Activity Handout. We provide physical and digital copies of this handout to each lab partner as instructions for the lesson.
- S2. Breaking Bricks – Introductory Slides for Enzyme Kinetics Activity. These slides provide introduction and guiding questions for the activity.
- S3. Breaking Bricks – Enzyme Kinetics Activity Datasheet. This sample spreadsheet provides one way to organize the data collected from the activity.
- S4. Breaking Bricks – Discussion Questions. These questions are assigned after the laboratory session and serve as a starting point for the second session's wrap-up conversation.
- S5. Breaking Bricks – Pre-Assessment. This anonymous survey was administered before the lesson and contains conceptual and graph interpretation questions about key concepts in enzyme kinetics and inhibition.
- S6. Breaking Bricks – Post-Assessment. This anonymous survey was administered after the lesson. It contains the same conceptual and graph interpretation questions as the pre-assessment, and also includes several attitudinal questions and a place for students to include comments and feedback about the lesson. A Google Form version of both assessments and an answer key are available on request.
ACKNOWLEDGMENTS
We would like to thank the instructors and students of BISC 220 in Spring 2018, 2019, and 2020 for providing feedback on several iterations of this lesson, Tess Killpack for assistance with survey data analysis, Leah Okumura for contributing to the original design of this experiment, and Steven Biller for photography. Funding for supplies to develop the lesson was provided by a Wellesley College Educational Research and Development grant to J.A.R.
References
- Alberts B. 2015. Molecular Biology of the Cell. W.W. Norton.
- AAAS. 2011. Vision and Change in Undergraduate Biology Education: A Call to Action., Washington, DC.
- Linenberger KJ, Bretz SL. 2015. Biochemistry students' ideas about how an enzyme interacts with a substrate. Biochem Mol Biol Educ 43:213-22. https://doi.org/10.1002/bmb.20868
- Rodriguez J-MG, Towns MH. 2019. Analysis of student reasoning about Michaelis-Menten enzyme kinetics: mixed conceptions of enzyme inhibition. Chem. Educ. Res. Pract. 20:428-442. https://doi.org/10.1039/C8RP00276B
- Rodriguez J-MG, Hux NP, Philips SJ, Towns MH. 2019. Michaelis-Menten Graphs, Lineweaver-Burk Plots, and Reaction Schemes: Investigating Introductory Biochemistry Students' Conceptions of Representations in Enzyme Kinetics. J Chem Educ 96:1833-1845. https://doi.org/10.1021/acs.jchemed.9b00396
- Freeman S, Eddy SL, McDonough M, Smith MK, Okoroafor N, Jordt H, Wenderoth MP. 2014. Active learning increases student performance in science, engineering, and mathematics. Proc. Natl. Acad. Sci. U.S.A. 111:8410-8415. https://doi.org/10.1073/pnas.1319030111
- Armbruster P, Patel M, Johnson E, Weiss M. 2009. Active learning and student-centered pedagogy improve student attitudes and performance in introductory biology. CBE Life Sci. Educ. 8:203-213. https://doi.org/10.1187/cbe.09-03-0025
- Forbes-Lorman RM, Harris MA, Chang WS, Dent EW, Nordheim EV, Franzen MA. 2016. Physical models have gender-specific effects on student understanding of protein structure-function relationships. Biochem Mol Biol Educ 44:326-335. https://doi.org/10.1002/bmb.20956
- Newman DL, Stefkovich M, Clasen C, Franzen MA, Wright LK. 2018. Physical models can provide superior learning opportunities beyond the benefits of active engagements. Biochem Mol Biol Educ 46:435-444. https://doi.org/10.1002/bmb.21159
- Bailey CP, Minderhout V, Loertscher J. 2012. Learning transferable skills in large lecture halls: Implementing a POGIL approach in biochemistry. Biochem Mol Biol Educ 40:1-7. https://doi.org/10.1002/bmb.20556
- Friedman EJ, Terry CH. 2020. Investigating enzyme structure and function through model-building and peer teaching in an introductory biology course. CourseSource. https://doi.org/10.24918/cs.2020.4
- Runge SW, Hill BJF, Moran WM. 2006. A Simple Classroom Teaching Technique To Help Students Understand Michaelis-Menten Kinetics. CBE Life Sci. Educ. 5:348-352. https://doi.org/10.1187/cbe.06-04-0160
- Hinckley G. 2012. A Method for Teaching Enzyme Kinetics to Nonscience Majors. J Chem Educ 89:1213-1214. https://doi.org/10.1021/ed200839v
- House C, Meades G, Linenberger KJ. 2016. Approaching a Conceptual Understanding of Enzyme Kinetics and Inhibition: Development of an Active Learning Inquiry Activity for Prehealth and Nonscience Majors. J Chem Educ 93:1397-1400. https://doi.org/10.1021/acs.jchemed.5b00562
- Junker M. 2010. A Hands-On Classroom Simulation To Demonstrate Concepts in Enzyme Kinetics. J Chem Educ 87:294-295. https://doi.org/10.1021/ed800082f
- Jacob F, Monod J. 1961. Genetic regulatory mechanisms in the synthesis of proteins. J Mol Biol 3:318. https://doi.org/10.1016/S0022-2836(61)80072-7
- Huber RE, Kurz G, Wallenfels K. 1976. A quantitation of the factors which affect the hydrolase and transgalactosylase activities of beta-galactosidase (E. coli) on lactose. Biochemistry 15:1994-2001. https://doi.org/10.1021/bi00654a029
- Juers DH, Hakda S, Matthews BW, Huber RE. 2003. Structural basis for the altered activity of Gly794 variants of Escherichia coli beta-galactosidase. Biochemistry 42:13505-11. https://doi.org/10.1021/bi035506j
- Lord T. 1998. Cooperative Learning That Really Works in Biology Teaching: Using Constructivist-Based Activities to Challenge Student Teams. Am Biolo Teach 60:580-588. https://doi.org/10.2307/4450554
- Moog RS, Spencer JN, Straumanis AR. 2006. Process-oriented guided inquiry learning: POGIL and the POGIL project. Metropolitan Universities 17:41-52.
- Hogan CF. 2003. Practical Facilitation: A Toolkit of Techniques. Kogan Page.
Article Files
Login to access supporting documents
Breaking Bricks: A Hands-On Model of Enzyme Kinetics and Inhibition(PDF | 602 KB)
S1.BreakingBricks-In-classActivity.docx(DOCX | 17 KB)
S2.BreakingBricks-IntroSlides.pptx(PPTX | 2 MB)
S3.BreakingBricks-EnzymeKineticsActivityDatasheet.xlsx(XLSX | 9 KB)
S4.BreakingBricks-DiscussionQuestions.docx(DOCX | 16 KB)
S5.BreakingBricks-Pre-Assessment.pdf(PDF | 254 KB)
S6.BreakingBricks-Post-Assessment.pdf(PDF | 293 KB)
- License terms
Comments
Comments
There are no comments on this resource.