Distances in the Universe: An Inquiry Lab Sequence Taught in West Africa and North America
Editor: Melissa Dancy
Published online:
Abstract
Astronomy evokes deep curiosity for many people, making it a beautiful topic for supporting students to learn scientific practices and develop as scientists. We present an inquiry-based lab sequence about distances in the Universe, which we have taught in a first-year astronomy course in Canada and in a summer program for upper-year students in West Africa. Students begin with two warm-up labs where they discover the methods of parallax and the inverse-square law for light to measure distances in their everyday lives. Then they engage in a mini research project in which they ask their own questions about astronomical images, then break down their big questions into smaller questions related to measuring astronomical distances. Students work together in teams to investigate their questions, and finally present their findings to their classmates. Students developing their own questions to investigate is a key scientific practice that is not included in many other inquiry lab curricula. We show evidence that students learned astronomical concepts, had positive feelings about the labs, appreciated the freedom to come up with their own approaches in the labs, and built their self-efficacy as scientists. Since facilitating inquiry is quite different from other kinds of teaching, we describe key features of our facilitation including how we teach new instructors. We describe our curriculum in both Canada and West Africa and offer suggestions for future implementations. We encourage other astronomy instructors to try an inquiry approach to help students develop as scientists while exploring topics they are curious about.
Primary Image: Students Rubby Aworka, Vincent Oko Laryea, and Mercy Effah Pardie (left to right) work on designing a way to measure the distance to a model planet in the Distance Inquiry Lab A, at the Pan-African School for Emerging Astronomers (PASEA) 2017 in Accra, Ghana. Image credit: Thai Duy Cuong Nguyen, provided with permission for use in this article under CC-BY-NC 4.0.
Citation
Strubbe L, Good D, Zhang J, White H, Lepo K, Code W, Abotsi‐Masters S. 2023. Distances in the Universe: An Inquiry Lab Sequence Taught in West Africa and North America. CourseSource 10. https://doi.org/10.24918/cs.2023.3Lesson Learning Goals
- Students will understand two important distance measurement techniques in astronomy.
- Students will be able to ask a scientific question about an astronomical phenomenon they are interested in, and design and implement their own plan to investigate it, without direct instruction from an authority.
- Students will be more likely to engage in scientific practices, particularly identifying and solving problems, in their other courses and future lives and work.
- Students will recognize and value that they are engaging in practices that are important for scientific work.
- Students will feel that they are developing as scientists.
- Students will recognize that many concepts and ideas in science are extensions or quantifications of ideas and methods they are already familiar with from their everyday lives.
- Students will value and expand their ability to work together in a team of fellow students.
Lesson Learning Objectives
Content:- Students will be able to explain how the method of parallax works, and use it to measure the distance to an object on campus.
- Students will be able to explain why the apparent brightness of an object decreases as the square of the distance from the object.
- Students will be able to use parallax or the inverse-square law for light to measure the distance to an astronomical object.
- Students will be able to identify circumstances when different techniques are appropriate for measuring distances to astronomical objects (parallax with Earth as a baseline, parallax with the Earth’s orbit as a baseline, inverse-square law with standard candle), and explain limitations of each technique.
- Students will be able to list important distance scales in astronomy.
- Students will be able to explain why measuring the distance to astronomical objects is critical to much of our knowledge about those objects.
- Students will understand how the cosmic distance ladder works: that each successive distance technique relies on measurements of closer distances.
- Students will be able to devise a question about an astronomical image that is investigable, with support from facilitators.
- Students will be able to take an investigable question and plan a path or procedure for investigating that question in a team, with support from facilitators.
- Students will feel more confident in their ability to identify and solve problems without an authority to rely on.
- Students will increase their self-identity as a scientist.
- Students will feel a sense of pride and accomplishment when overcoming their challenges in the labs.
Article Context
Course
Article Type
Course Level
Bloom's Cognitive Level
Class Type
Class Size
Audience
Lesson Length
Pedagogical Approaches
Principles of How People Learn
Assessment Type
Introduction
“There are model planets hanging in this field. How can we figure out how far away they are… without being able to go to them? This is a major problem we face in trying to learn about planets in space! Your Challenge: Determine the distance to the model planet, without going past this concrete strip.”
This prompt is how we started our sequence of inquiry-based labs in Astronomy 101 at the University of British Columbia (UBC) in Fall 2017. In the world of physics labs, the American Association of Physics Teachers (1) recommends learning outcomes that focus on scientific practices: e.g., constructing knowledge and designing experiments. They add that “the laboratory curriculum should get students to start thinking like physicists by constructing knowledge that does not rely on an outside authority, should explicitly make them aware that they can construct knowledge in this way, and should build confidence in their ability to do so.” Wieman (2) and others discuss similar themes. While experimentation looks different in astronomy compared to physics, these ideas are relevant for astronomy labs as well.
Inquiry is a powerful approach to teaching scientific practices intertwined with scientific content (e.g., [3]). Inquiry has a diversity of definitions, but the essence is “engaging students in the thinking processes and activities of scientists” (4). Astronomy is an inspiring topic for inquiry labs because many students have curiosity about space and the Universe. At the same time, astronomy can be a challenging arena for developing scientific practices, since it is an observational (rather than experimental) science, and most astronomical observations require specialized equipment and/or precious telescope time. Ways forward include students interpreting astronomical data (without taking the data themselves), creating physical or computer models of astronomical phenomena, experimenting with optics, and designing a telescope (e.g., [5–8]). In our lab sequence, students design the type of observation they want for their investigation, then are given the relevant (archival) data (and/or simulated data, simplified for easier analysis) once they‘ve described the hypothetical observation process carefully.
The topic of our inquiry lab sequence is “Distances in the Universe.” We chose this content area for several reasons: (i) Knowing the distances to astronomical objects underlies so much of what we know about the Universe, from object sizes to energy scales and cosmological expansion rates; (ii) The basic techniques of measuring distances in space have everyday analogues we use in our everyday lives; and (iii) Recognizing the vast distance scales in the Universe often inspires awe. Concepts around astronomical distance and their measurement thus comprise an “enduring understanding”—a high curricular priority according to the principle of backward design (9). These reasons also make the topic appropriate for diverse astronomy education contexts.
We gratefully acknowledge inspiration for Lab A from parallax labs by D. V. Black and Ladd et al. (10) / E. F. Ladd, personal communication (2017), and inspiration for our Lab B from inverse-square law labs by the Exploratorium Teacher Institute and Structure and Evolution of the Universe Education Team. The cited labs are traditional lab curricula and/or demonstrations where students are given the step-by-step procedures and methods of analysis. In our inquiry warm-up Labs A and B, students make measurements and calculations similar to those in the cited labs (Lab A: using parallax to measure the distance to a model astronomical object; Lab B: comparing areas of light at different distances from the source), but they also develop their own procedures, theoretical ideas, and methods of analysis to get there. Another difference is that in our Lab B, students primarily compare the sizes of shadows; in the cited inverse-square law labs, students compare the sizes of projected squares of light.
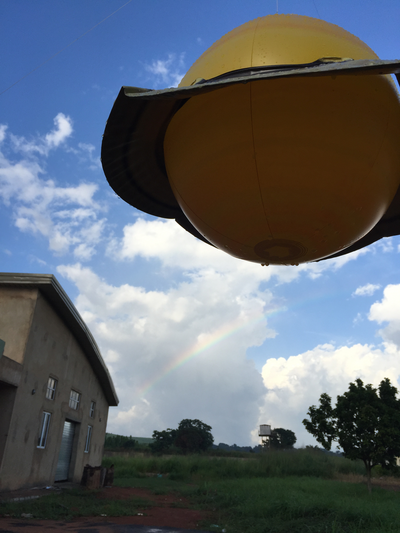
In this article, we share our inquiry lab curriculum so that other educators may try something similar or be inspired to develop something new. We include descriptions of our facilitator development for teaching assistants (TAs) and our assessments of student learning. Our full set of curriculum documents is available in the Supporting Materials. LS, KL, HW and JZ originally designed this inquiry activity in 2013 for the Pan-African School for Emerging Astronomers (PASEA; see [11]) as part of the Institute for Scientist and Engineer Educators (ISEE) Professional Development Program, with further contributions by colleagues listed in the Acknowledgements.
This article’s main focus is our adaptation of the PASEA activity for the lab component of “Introduction to the Solar System,” a first-year astronomy course at UBC, a course with about 100 students. Most students in this course were majoring in science but not physics or astronomy. Each week, students had one two-hour lab and three one-hour lectures; the labs and lectures were largely decoupled. Each lab section contained about 20 students taught by two facilitators (an instructor or lead TA, and a newer TA). The inquiry labs comprised five out of nine total labs in the course. The other four labs were hands-on but not full inquiry; the topics were the Sun, the cratering process, Pluto, and exoplanets. These labs were independent from the inquiry lab sequence discussed in this article. Bringing inquiry to the labs was part of a department-wide effort to transform courses to active learning: the lectures were transformed the previous year. LS was a Science Teaching & Learning Fellow (STLF) and led the inquiry curriculum design and facilitation, in close collaboration with DG who was the Inquiry TA and led the TA development. The Lesson Plan description, Supporting Materials, and Evaluation of student learning (in the Teaching Discussion) refer to the inquiry lab sequence at UBC. We describe the inquiry activity at PASEA later in the Teaching Discussion.
Intended Audience
We have taught this curriculum for two populations of students: first-year science (non-physics / non-astronomy) majors at a large research university in North America, and upper-year science students interested in astronomy at a summer program in West Africa.
Required Learning Time
The presented curriculum is designed for five weekly two-hour lab sessions with a few hours of homework each week (typically 0–1 hour for each pre-lab and 1–3 hours for each post-lab assignment). It has also been taught as a twelve-hour block with no homework, spread over three days at a summer program.
Prerequisite Student Knowledge
-
Basic algebra (ratios, solving linear single-variable equations), scientific notation, converting between units, working with exponents.
-
Basic trigonometry: sides and angles of a right triangle, radians and degrees, complementary angles, and the small-angle approximation; reviewed in S3 and S6.
Prerequisite Teacher Knowledge
Some of these concepts are also outlined in the Supporting Materials.
-
How to facilitate inquiry. We describe our approach in the Lesson Plan (see Inquiry facilitation and facilitator development for teaching assistants subsection).
-
How the method of parallax works for measuring distances in astronomy, for Solar System objects (size of Earth is the baseline) and nearby stars (orbit of Earth is the baseline), including the need for a background reference object, and limitations due to minimum measurable angular resolution.
-
How to use the distance from the Earth to Venus during a transit of Venus to calculate the distance from the Earth to the Sun.
-
Why the inverse-square law for light is true (see, e.g., Wikipedia’s article on inverse-square law)
-
The difference between apparent and intrinsic brightness.
-
What standard candles are and how to use them with the inverse-square law for light to measure distances.
-
How to identify the spectral type of a main sequence star and infer the star’s luminosity (matching to a given set of spectra and listed luminosities).
-
What the cosmic distance ladder is.
-
(Optional) How to use the inverse-square law for light with Cepheid variable stars and Type Ia supernovae to measure distances to extragalactic objects.
Scientific Teaching Themes
Active Learning
Our labs are based on an inquiry paradigm from the Institute for Inquiry at the Exploratorium and ISEE, which draws from Chinn & Malhotra’s theoretical framework for authentic inquiry tasks (12). In this paradigm, inquiry activities comprise three stages: (i) Engaging the learner, (ii) Focused investigation, and (iii) Making meaning together (13). These stages take learners through a path of a mini research project working in a small group, from asking their own questions to developing their own investigation plan to synthesizing and sharing their results with their classmates. Learning from Inquiry in Practice (14) includes several astronomy inquiry activities following the ISEE / Exploratorium paradigm.
Various curricula for physics / astronomy called “inquiry” (in publications and/or informally) show significant benefits for student learning (with the caveat that the associated studies likely focus on a narrow subset of students, as do most studies in physics education research; [15]). For example, the Investigative Science Learning Environment (ISLE) approach emphasizes students’ creating and testing their own explanations for everyday phenomena, as scientists do. ISLE students learned concepts and designed experiments in new contexts more effectively than students in traditional classrooms, and they appreciated figuring things out on their own and working in groups (16). Holmes, Keep & Wieman (17) showed that a physics lab curriculum that provides scaffolding for students to make decisions in designing their experimental procedures (rather than making decisions for students) and prompting students to evaluate or compare models (rather than verify a particular physics result) supported students’ decision-making and epistemic agency. Similar labs brought students to more expert-like engagement with experimentation and attitudes about experimental physics (18, 19). Slater et al. (20) created a “backwards faded scaffolding” approach to astronomical inquiry, sequentially shifting responsibility for experimental tasks from instructor to students over the course of five inquiry phases; their students showed significant gains on an astronomical concept inventory and increased their self-identity as scientists.
Because our students ask their own questions, Buck et al.’s rubric (21) would characterize our lab sequence as “authentic inquiry,” the highest level. We note that many lab curricula referred to as inquiry (in publications and/or informally) do not include the scientific practice of students asking their own questions (e.g., Physics by Inquiry [22, 23]; ISLE [16, 17]); Slater et al.’s curriculum intentionally builds to this practice but reaches it only in their last inquiry phase (20). For us, asking questions is a crucial practice to include in inquiry, to support students’ agency, ownership, creativity, and views about science. Building space for students to ask their own questions demonstrates our belief that not only can students figure out answers for themselves, but also that students can ask interesting questions, and do not have to rely on an authority to tell them what questions are worth investigating. We believe this is empowering, motivating, and truer to the nature of scientific research (and also, not easy to do!). We describe how we support students in this process in the Lab C: Questions subsection within the Lesson Plan.
Because inquiry invites students to make a full cycle of scientific investigation, we expect that inquiry can be particularly strong for helping students develop scientific attitudes and strengthening their understanding of the nature of science. Several common surveys exist for measuring these constructs in different science fields; e.g., the Views of the Nature of Science Questionnaire (24) and Colorado Learning Attitudes about Science Survey (CLASS: [25]). In physics, instruction with a focus on collaboratively developing models of the physical world has been shown to develop students’ attitudes towards those of experts, when active learning alone does not (26, 27). In biology, inquiry labs have been shown to help students gain confidence and develop attitudes about science that are closer to those of experts, relative to interactive lectures alone (28). We share our CLASS results at UBC and PASEA in the CLASS subsection and the Inquiry activity at the Pan-African School for Emerging Astronomers (PASEA) subsection of the Teaching Discussion (respectively). Other studies show the benefits of inquiry compared with traditional lecture (e.g., [29] in chemistry), in some cases also improving equity (e.g., [30, 31] in math). Note that the definitions of inquiry vary across these studies; indeed in some studies, the term and results for “inquiry” are not clearly distinguished from “active learning” and/or “student-centered teaching”; e.g., (23). Inquiry is beneficial for teaching science at K-12 levels as well (e.g., [3]). We add the caveat that most research on inquiry (and science education more broadly) explores learning among students in North America; it is important that future research focus further on how students from educational and cultural backgrounds beyond North America learn science.
Our lab sequence aligns well with Lombardi et al.’s definition of active learning as “a classroom situation in which the instructor and instructional activities explicitly afford students agency in learning” (32): our students ask their own scientific questions and design and carry out their own investigations, with curricular scaffolding and support from instructors. Our labs also align with a number of Lombardi et al.’s recommendations (32), e.g., affording the social construction of understanding with peers (students working in small groups); addressing and supporting positive emotional aspects of learning (enjoyment, pride); and including student engagement with direct experiences of phenomena (parallax, inverse-square law for light), scientific models, and scientific data.
Assessment
We use a variety of modes to measure students’ learning. Informally, facilitators gauge students’ progress during the labs by observing students’ writing and drawing, listening to students’ group conversations, and occasionally asking students questions. For more formal assessment, students submit pre-lab and post-lab assignments for each lab in the sequence. After each lab, students write open responses to several reflection questions about what they learned in the labs, what went well in their approach, what questions they still have about the topic, etc. At the end of the lab sequence, students demonstrate and share their learning via a group presentation and an individually written lab report. As part of the lab report, students are prompted to reflect on their strategies for problem solving, how their team worked together, challenges that arose and how they overcame them, and what they learned about being a scientist. There were also a few questions related to distance measurement on the course midterm and final exams. For a typical implementation of this lab sequence, we would recommend the assessments listed in this paragraph.
Because we were implementing this lab sequence for the first time, as part of a department-wide effort in course transformation, and imagining that we might want to publish our curriculum in the future, we conducted additional assessments that would likely be too much for a typical implementation. We assessed students’ learning and impressions of the labs in aggregate through anonymous pre- and post-semester surveys about students’ attitudes (CLASS: [25]), self-identity as a scientist (adapted from [33]), and (post-only) perspectives on helpfulness of different course components. Students self-reflected on their learning via survey questions following each lab (graded for completion): for nine scientific practices, students selected which they engaged in during the lab, which they found personally interesting, and which they felt they got better at. Students also responded via a Likert scale (34) about their feelings following the lab (e.g., feeling confident, confused, proud), and wrote open responses to reflection questions (as mentioned in the previous paragraph, and listed later in this article).
Inclusive Teaching
We describe our inclusive teaching practices through ISEE’s equity framework (35). Our curriculum is designed to support success for diverse students by providing multiple ways to participate productively. Students express their ideas in diverse ways, through group discussion, writing equations and words, drawing, working with physical models, and an oral presentation. The sequence includes components where students work alone and in groups. Facilitators pay close attention to group dynamics to make sure that each student is being heard and understands. The curriculum is designed to connect to learners’ own goals, interests and values, by inviting students to ask their own questions they are curious about in astronomy, and encouraging students to come up with their own ways of thinking about the concepts. Connected to this, the curriculum helps students develop their identities as people in STEM, which typically aligns with their career goals as STEM majors. Students are prompted multiple times to reflect on their scientific practices (e.g., asking questions, designing investigations, working in teams), and what they are learning about being a scientist, to highlight that their work in the labs is helping them to develop as scientists. Last, the curriculum is designed to support positive beliefs about learning, achievement and teaching, which can lead to higher self-efficacy. The labs include scaffolding to help students work through challenges: e.g., students learn to break down big questions into smaller ones, and work together in groups. Facilitators are asked to interact with students in line with the assumption that students are curious, motivated, and able to succeed. The lab report assignment prompts students to reflect on challenges they faced and how they overcame them. Working together on a challenging problem that (likely) no student could solve alone also highlights that each student has diverse and valuable contributions to make. The topic of the cosmic distance ladder, in which each successively far distance measurement relies on closer measurements, also highlights the interdependence and collaborative nature of scientific research.
Lesson Plan
Concept background
The two primary concepts that astronomers use in measuring distances in the Universe involve parallax and the inverse-square law for light (ISL), defined as follows. Parallax: if we measure the apparent position of a target object (e.g., Solar System planet) relative to a distant reference object (e.g., distant star) while standing at two different locations, and also the physical distance between those locations, we can use trigonometry to calculate the distance to the target object. Because of our limited ability to resolve tiny angular differences, parallax can only be used to measure distances to objects relatively close (much less than the size of our Galaxy). ISL: The apparent brightness (flux) received from an object decreases as the inverse-square of its distance away. Thus, if we observe two of the same type of object at different distances, and we know the distance to one of the objects (e.g., via parallax), we can calculate the distance to the other. A challenge in astronomy is to identify that two objects are of the same type, i.e., have the same or related intrinsic brightness; this is typically done by comparing spectra and/or light curve periods and shapes. (For the PASEA version of the labs where we include extragalactic objects, we simplify that all Type Ia supernovae have the same intrinsic peak brightness.) Astronomers build out our knowledge of distances to ever-further objects in what is called the “cosmic distance ladder”, where techniques used to measure close-by distances provide information (e.g., the size of the Earth’s orbit, the intrinsic brightness of the Sun) that are used in techniques for measuring further distances. The Wikipedia article on the Cosmic Distance Ladder provides a nice summary. (Note that currently radar is used to measure precise distances in the Solar System. However, because this is conceptually quite simple, if students propose to use radar, we encourage them to explore it briefly but then to consider how this measurement could be done / was done prior to the invention of radar.)
The lab sequence is divided into five two-hour lab sessions (A–E), most of which have an associated pre- and post-lab assignment. Labs A and B are “warm-ups” that respectively introduce students to the concepts of parallax and inverse-square law for light, and the exploratory style of these labs. Labs C–E are the inquiry cycle, comprising asking questions, planning, and carrying out an investigation, and sharing results with classmates. In the Supporting Materials for each lab (Tables 12A and B), we provide the Lesson Plan; TA meeting plan; slide deck (if applicable); and pre-lab, in-lab, and post-lab assignments with answer keys and rubrics. Tables 1–3 and 6–8 show the recommended timeline for the lab sequence.
Table 1. Lesson plan timeline for the beginning of the semester.
Activity | Description | Timing | Associated Documents |
---|---|---|---|
Facilitator Development Session (S52. Pre-semester TA Meeting Plan) | |||
Before session | |||
Pre-reading | Participants read in preparation for the session | ~1 hour |
Inquiry-based learning (L. Strubbe 2014) Facilitating the focused investigation (Exploratorium 2005) This current CourseSource article S56. Inquiry labs overview - for students |
Lab preparation |
Print starter images of astronomical objects (only Solar System parallax) and hang up around the lab room Hang poster paper around the lab room |
~15 min | S35. Lab C - Starter images |
During session | |||
Introduction | Welcome and overview of the session | 0:00–0:05 | S52. Pre-semester TA Meeting Plan |
Participants do inquiry activity | Participants do abbreviated version of the inquiry lab sequence (Labs C and D, only Solar System parallax investigation path), pretending to be students | 0:05–2:00 |
S26. Lab C - Lesson Plan S36. Lab D - Lesson Plan Facilitation guides and data for Parallax Type 1: S58. Facilitation guide (short version) S59. Facilitation guide (full version) S60. Description of the simulated data |
Discussion | Participants reflect on inquiry experience, discuss facilitation strategies, ask questions | 2:00–3:00 |
S53. Facilitator reflection prompts S54. Notes for grading inquiry labs |
For students (e.g., in lecture component of the course) | |||
Introduce inquiry to students | Briefly discuss inquiry labs overview during first week of semester to prepare students | ~5 min | S56. Inquiry labs overview - for students |
Lab A: Model planets inquiry warm-up
The overall goals of this lab (S1) are for students to discover and use parallax for measuring distances, and practice working in small groups. Students begin with an online prelab assignment (S3) to remind them of simple trigonometry and other concepts needed for the lab.
This lab takes place outside. We find an open area on campus with a long sidewalk (which represents the surface of the Earth), and hang inflatable model planets in a field (which represents space) in front of the sidewalk, making sure there are landmarks (like towers or signposts) that can serve as reference points: see Figures 1 and 2. We give students the challenge of finding a way to measure the distance to a model planet without crossing beyond the sidewalk (i.e., without leaving the surface of the Earth). We have tools available such as chalk, tape measure and protractors, but without telling students they must use any of these or how they should use them. Students work in groups of four to brainstorm, write down ideas, and try things out. Students choose their own [possibly different] groups for Labs A and B, though an instructor could create the groups instead if there is reason to do so. We make sure that all groups come up with some variation on the concept of parallax, either on their own, or with some gradual hints from facilitators. We have “hint sheets” (see Figure 2) with incomplete diagrams and additional question prompts that we offer to groups when they seem stuck or in need of new inspiration. Eventually, each group draws their own diagram, chooses their measurement tools, makes measurements (length of baseline and an angle), and calculates the distance to a model planet. The short video “WAISSYA 2017: How far away is the planet?” by Inigo Films shows students engaging in a variation of Lab A at PASEA 2017 in Accra, Ghana.
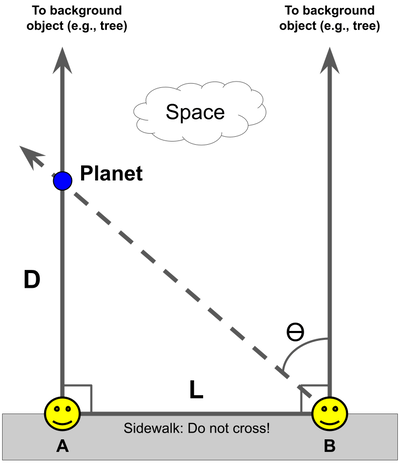
Note that in Lab A and all labs in this sequence, we did not require students to use appropriate uncertainties because we chose to focus on other scientific practices, and because most students were concurrently taking a physics lab course that teaches uncertainty analysis. An instructor could choose to include uncertainty analysis as part of this lab sequence.
Students submit a worksheet in which they describe their method (S4). Interestingly, we saw that our students came up with a variety of ways of doing parallax, including ways we hadn’t thought of before. This demonstrates that the students used their minds to create their own methods, in contrast to other labs in which they implement a method taught by an authority. Then students work on a follow-up worksheet (starting in lab, finishing online at home; S8) to practice and further extend the concept of parallax for measuring distances, including recognizing that parallax does not work for objects that are so far away that the difference in angular position cannot be measured within uncertainties. The post-lab assignment (S8) includes a section (S55; graded for completion) where students reflect on their learning in the lab (see the Students’ reflections on their learning subsection in the Teaching Discussion), scientific practices they engaged in, and how they felt at the end of the lab (see the Students’ feelings about their experiences in the labs subsection in the Teaching Discussion).
Table 2. Lesson plan timeline for Lab A - Model planets warm-up. (S1. Lesson Plan)
Activity | Description | Timing | Associated Documents |
---|---|---|---|
Before lab | |||
Lab preparation |
Select outdoor location for the lab Hang model planets Draw a line on the ground Try out the lab in selected location Gather equipment Print worksheets, hint sheets, trig tip sheets Post pre-lab assignment on LMS Post post-lab assignment on LMS (hidden until after lab) including survey questions |
~2 hours |
S1. Lesson Plan S3. Pre-lab Questions S4. In-lab worksheet S5. In-lab hint sheets S6. In-lab Trig Tip sheet S7. Post-lab - Worksheet version S8. Post-lab - Version to post on LMS S55. Questions for students after labs A, B, E |
Facilitator development session | Facilitators learn how to facilitate Lab A | ~1.5 hours | S2. TA meeting plan |
In lab | |||
Introduction | Introduce the inquiry lab sequence, introduce the model planets challenge, hand out worksheets, students get into groups, give advice for working together productively | 0:00–0:05 | S1. Lesson Plan |
Students work on model planets challenge |
Students work in groups on the model planets challenge to discover the method of parallax. Hand out Hints A, B, and C to groups when they get stuck or need some extra support. Suggested around 0:25, 0:40, and 1:05. |
0:05–1:25 |
S4. In-lab worksheet S5. In-lab hint sheets S6. In-lab Trig Tip sheet |
Students start post-lab assignment | Hand out post-lab worksheet. Students start work on this worksheet version, now and continue at home with LMS version. | 1:25–1:40 |
S7. Post-lab - Worksheet version S8. Post-lab - Version to post on LMS |
Wrap-up | Congratulate students on their hard work, summarize concepts we aimed for them to learn | 1:40–1:45 | |
After lab | |||
Grading |
Grade Pre-labs Grade In-lab worksheets Grade Post-labs Post pre-lab answers (optional) Post post-lab answers (optional) |
Depends on number of students |
S9. Pre-lab Answers S10. In-lab worksheet - Rubric S11. Post-lab Answer key and rubric S12. Post-lab Answer key to share with students |
Lab B: Light and brightness inquiry warm-up
Lab B (S13) is to help students discover the inverse-square law for light.
In our experience, almost all students intuitively recognize that more distant objects appear fainter. Students often guess that the relationship between distance and apparent brightness is inverse-linear rather than inverse-square; some students have been taught the inverse-square law, but do not have an intuitive understanding of where this comes from. This lab is to help students discover and build a mental model of why the relationship is inverse-square.
Students complete a pre-lab assignment (S15) to review basic geometry of circles and spheres and review the idea of light as flowing (like water). In the lab, students begin with an invention activity using contrasting cases (in the style of [36]) about the difference between absolute and apparent brightness (see S13). We switch on a globe light and ask students to discuss in groups, “Does the brightness of the bulb depend on how far you are away from it?” After a period of small-group discussion (without facilitators giving any “answers”), we prompt students to invent their own definition (or definitions) of brightness, including what units it should have. After further discussion, facilitators announce (but do not explain in detail) that many groups have figured out that there are actually two phenomena we informally call “brightness”: absolute (or intrinsic) brightness which does not depend on distance, and apparent brightness which does.
With these partially formed ideas in mind, students continue to develop their understanding through working on two related challenges. In the first (on paper; S16), students are asked to create and explain an equation that relates distance from the Sun with the power received by a solar panel of fixed area, in the context of an expedition to the dwarf planet Sedna. In the second (S17), students are given a light bulb to play with, and challenged to create an equation to calculate the sizes of shadows (created by their hands, cardboard squares, anything they choose). Students have rulers and light-meters available to measure areas and flux levels if they choose. There is an optional side experiment (S18) where students can use an apparatus to observe the spherical expansion of light into concentric shells, and another hint worksheet (S19; Figure 3) to help students draw expanding rays of light. There is a post-lab assignment (S21) to synthesize what students learned about the inverse-square law, and the same reflection questions as in Lab A (S55).
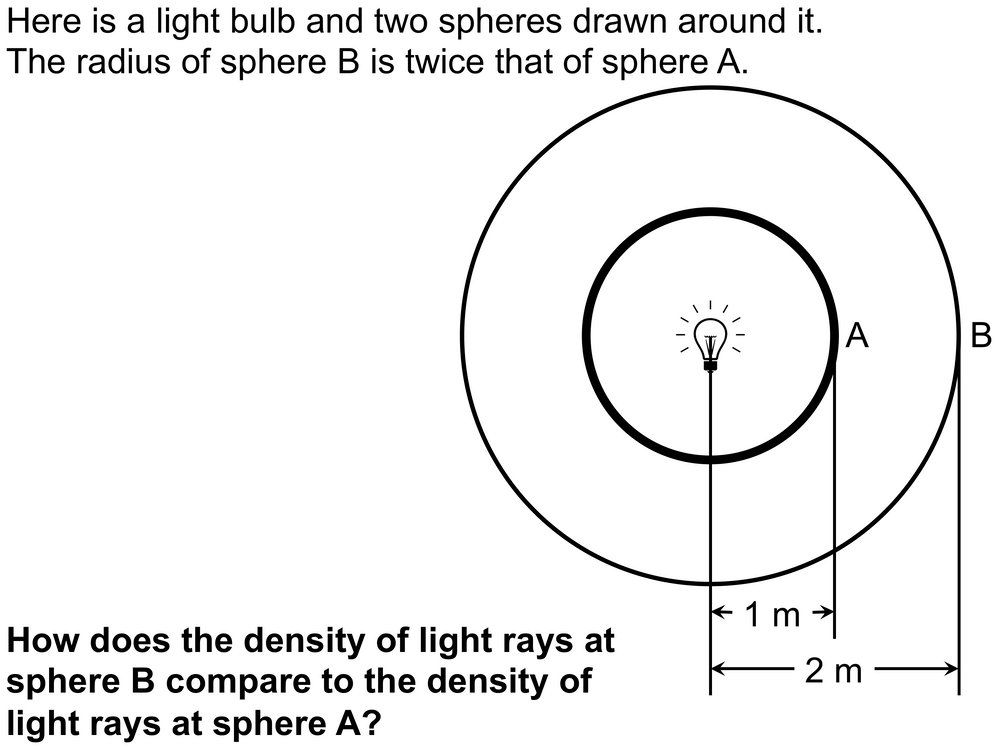
Table 3. Lesson plan timeline for Lab B - Light and brightness warm-up. (S13. Lesson Plan)
Activity | Description | Timing | Associated Documents |
---|---|---|---|
Before lab | |||
Lab preparation |
Build hemispheres apparatus Test out experiment Gather equipment Print worksheets Distribute poster paper and markers to each group table Post pre-lab assignment on LMS (without answers) Post post-lab assignment on LMS (hidden until after lab) including survey questions |
~3 hours |
S13. Lesson Plan (this doc includes instructions for building hemispheres apparatus in the Appendix) S15. Pre-lab (with answers) S55. Questions for students after labs A, B, E |
Facilitator development session | Facilitators learn how to facilitate Lab B | ~1.5 hours | S14. TA meeting plan |
In lab | |||
Introduction | Introduce the lab, hand out worksheets, students get into groups | 0:00–0:05 | S13. Lesson Plan |
Invention activity |
Students work on invention activity about what brightness means Give hint after 10 minutes |
0:05–0:25 | |
Students work on Inverse-Square Law problems |
Hand out Sedna worksheet Students work on Sedna worksheet After ~15 minutes, hand out Shadow Puppets worksheet and demonstrate. Students work on Shadow Puppets worksheet. Offer optional Thinking Tools on Hemispheres and Light Rays to groups as appropriate. Suggested around 1:05 and 1:15. |
0:25–1:40 |
S16. Worksheet 1 - Sedna S17. Worksheet 2 - Shadow puppets S18. Worksheet 3 - Hemispheres S19. In-lab Light rays hint sheet S20. In-lab List of constants |
Wrap-up | Congratulate students on their hard work, summarize concepts we aimed for them to learn, remind students to work on post-lab assignment | 1:40–1:45 | S21. Post-lab |
After lab | |||
Grading |
Grade Pre-labs Grade In-lab worksheets Grade Post-labs Post pre-lab answers (optional) Post post-lab answers (optional) |
Depends on number of students |
S22. Worksheet 1 - Sedna - Answer Key S23. Worksheet 2 - Shadow puppets - Answer Key S24. Worksheet 3 - Hemispheres - Answer Key S25. Post-lab Answer key and rubric |
Labs C, D and E: The inquiry cycle
The lab sequence is structured to offer a variety of possible investigation paths, all of which relate to measuring the distance to a celestial object. The objects and associated distance measurement techniques are listed in Table 4. There are three types of investigation paths, depending on the method needed for measuring the distance: (i) Solar System objects, which use parallax where the baseline is two separated locations on Earth (at the same time; see S58 and S59); (ii) nearby stars and associated exoplanets, which use parallax where the baseline is the Earth at points on its orbit six months apart (see S61); and (iii) a distant star, which uses the inverse-square law for light with the Sun as reference star (see S71). In the PASEA version of the lab sequence, we also include the investigation paths: (iv) nearby galaxies, which use the inverse-square law for light with Cepheid variable stars as the reference objects; and (v) distant galaxies, which use the inverse-square law for light with Type Ia supernovae as the reference objects. Within the same inquiry lab structure, instructors can also create other investigation paths with other data, with related but different learning goals and/or in response to their students’ particular interests.
Table 4. Overview of the distance-related phenomena students can investigate in the inquiry cycle Labs C–E. These are the astronomical objects, associated distance measurement techniques, and examples of investigable questions students may ask that ultimately motivate them to find the distance to their chosen object. See also S40 for distance answers, and S58–S75 for facilitation guides and simulated data for each investigation path. Note that the curriculum documents we are sharing with this article do not include the additional extragalactic objects in the PASEA version of the labs, but interested instructors can create materials for these investigation paths following the same format as the others.
Object | Distance Method | Data Needed | Example Question(s) |
---|---|---|---|
Moon | Parallax |
Phenomenon that enables measurement: lunar occultation of a star Baseline: different locations on Earth at about the same time Reference background object: the star Data we provide: diagram of lunar occultation of Regulus observed from many cities, map showing locations of the cities |
What are those dark patches on the Moon? |
Geometry (not parallax) |
Phenomenon that enables measurement: lunar eclipse Data we provide (or students may know or look up): duration of lunar eclipse, radius of the Earth, orbital period of the Moon |
||
Venus | Parallax |
Phenomenon that enables measurement: Transit of Venus across the Sun Baseline: different locations on Earth at about the same time Reference background object: the Sun Data we provide: simulated image of the Sun during the transit of Venus in 2012 from Anchorage, Alaska & Sydney, Australia Students can also use Stellarium to “observe” the precise location of Venus or other Solar System objects from widely separated locations on Earth. |
How long did it take the Pioneer spacecraft to reach Venus? How big are the cloud features on Venus? |
Sun | Parallax |
Same as for Venus plus: Phenomenon that enables measurement: Observe phases of Venus Data we provide: Angular separation between Venus and the Sun when the phase of Venus is first/last quarter. |
How tall are giant solar prominences? |
Other Solar System planets | Parallax |
Same as for Sun plus: Phenomenon that enables measurement: Observe that planets’ orbital periods follow Kepler’s 3rd Law (ratio version) Data we provide: Orbital periods of planets |
How did the Caloris Basin on Mercury form? |
Nearby stars and exoplanets: 51 Pegasi, Fomalhaut B debris disk, Proxima Centauri (and exoplanet), Gliese 445 | Parallax |
Phenomenon that enables measurement: Earth orbits the Sun Baseline: Location of the Earth in its orbit six months apart Reference object: Distant stars Data we provide: Pair of images of object observed 6 months apart, angular size of pixels in the images |
How big is the Fomalhaut B debris disk? How long will it take Voyager 1 to reach Gliese 445? |
The Earth-sized exoplanets Kepler-20e and Kepler-20f | ISL |
Phenomenon that enables measurement: All (main-sequence) stars of the same spectral type have the same intrinsic luminosity Data we provide: Kepler 20 spectrum; reference spectra for one example of a star from each spectral type (OBAFGKM), with the distance (which we tell students another group is measuring using 6-month parallax) and measured flux from each reference star; Kepler 20 light curve (so students can calculate orbital radius of planet after calculating intrinsic brightness of star, e.g., for determining if liquid water could be present). |
How long would it take for us to receive a signal from or travel to Kepler-20e? Could there be life on Kepler-20e? |
Additional objects in PASEA version of the labs | |||
Nearby galaxies | ISL |
Phenomenon that enables measurement: The period of a Cepheid variable star is related to its intrinsic brightness Data we provide: Light curve (including flux) from a Cepheid variable star in the target galaxy; data for a set of Cepheid variable stars that all have the same period (their light curves, fluxes and distances, so students can discover that they all have the same intrinsic brightness); data for the period-luminosity relation for Cepheid variable star. We tell students that the distances to the reference Cepheids are measured using 6-month parallax, which another group is investigating. |
Why do galaxies have spiral structure? How does this galaxy compare to the Milky Way? |
Distant galaxies containing Type Ia supernovae: M101 and NGC 3147 | ISL |
Phenomenon that enables measurement: All Type Ia supernovae have the same peak brightness (students discover this from data we provide) Data we provide: Light curve of a Type Ia supernova in the target galaxy, light curves for a set of reference Type Ia supernovae, distances to the reference supernovae (which we tell students another group is measuring using Cepheid variable stars) |
What is that bright spot in the galaxy? |
Lab C: Questions
In Lab C (S26), we hang images of astronomical objects with short captions (list of captions in Table 5; example shown in Figure 4; printable slides in S35) around the room, with poster paper and markers beside each. Students are asked to visit each image and write down questions that interest them. To help inspire questions, we encourage students to discuss with others what features of the images stand out, and what they are curious about. Since classes so often require students to give very specific responses, we don’t want students to artificially shut down areas of interest, which can be de-motivating and lead to only a narrow set of questions. We encourage students to let their ideas flow openly and creatively, and not to censor themselves. We tell them no question is too easy or too hard, and not to answer their own or anyone else’s question during the brainstorming.
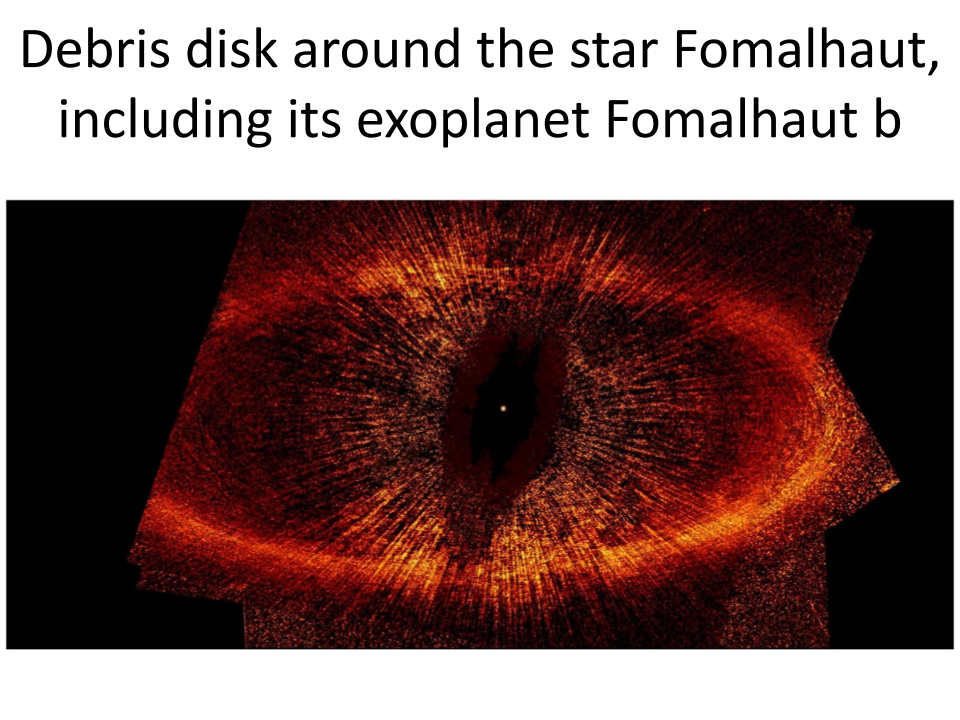
Table 5. List of astronomical images with captions that we hang around the lab room to inspire students’ questions during Lab C (S35). Note that the curriculum documents we are sharing with this article do not include the additional extragalactic objects in the PASEA version of the labs, but appropriate images can be easily found online.
List of astronomical images |
---|
|
Additional extragalactic objects in PASEA version of the labs |
|
Our intention is that many of the questions students ask will connect to distance, so that their question leads them to make a distance measurement. As mentioned, many astronomical questions involve distance, including those related to intrinsic brightness, size, and time to travel to the object. Questions like, “What is X’s feature?” typically can break down to include, “What are the physical characteristics of the feature?”—which includes its size and thus leads to distance. The questions students ask that do not connect to distance are typically those related to temperature and composition (which use color and spectra), movement (which may use Doppler shift or time-separated observations of proper motion), and optical properties of images (e.g., diffraction spikes). If students are not asking enough distance-related questions, facilitators can gently prompt those types of questions while students are discussing.
Following the questioning phase, facilitators take down the posters, read through all the questions and circle those which they deem appropriate for investigations in this lab, as above. It is important to commend all questions and explain why not all questions are selected: some are not connected to our content learning goals for the lab sequence, and some require time, materials, or background knowledge beyond what we have available. We let students know that their investigations will likely not produce new knowledge in the world, but that it is valuable to practice figuring out something that is new to them and learn where ideas come from. Thus, in this lab they will work to figure out everything from primary observations, without looking up numbers or ideas online or in books (S34).
In the next phase, students review the circled questions, and form small groups (3–4 or possibly 5 students) around a question they are all interested in investigating. Then students work together in their groups to break their bigger challenging question into smaller sub-questions, which should include some related to distance. Facilitators guide students to focus on an investigation related to distance, and students begin brainstorming how they might proceed in their investigation.
Note that it’s important to set expectations appropriately and facilitate this part carefully, as this phase moves from encouraging students to ask any question they can think of to narrowing the set of questions that students can investigate in the lab. This can be tricky, because we want students to feel that they have agency to ask freely and choose a question to investigate that they are interested in, yet we also have a (somewhat hidden) agenda that students will end up working on a distance-related sub-question, since many astronomical questions are in some way connected to distance. We explain to students that the narrowing is to help them choose questions they would be able to answer with the time and data resources available. To students who ask for more justification, we add that there are particular skills and concepts that we wanted them to focus on in the context of the course. Feedback from most students indicates they understand this approach, although at least one student expressed a negative impression that the facilitators were not being transparent. We encourage instructors to make this transition feel more open.
In the post-lab assignment (S30), students describe their team’s process for beginning to plan their investigation and think of another situation in their lives where they have broken down a bigger challenging question into smaller sub-questions.
Table 6. Lesson plan timeline for Lab C - Asking questions. (S26. Lesson Plan)
Activity | Description | Timing | Associated Documents |
---|---|---|---|
Before lab | |||
Lab preparation |
Print starter images of astronomical objects (from slide deck) and hang up around the lab room Hang poster paper around the lab room Print In-lab worksheets Post pre-lab assignment on LMS Post post-lab assignment on LMS (hidden until after lab) |
~30 min |
S26. Lesson Plan S28. Pre-lab S29. In-lab worksheet S30. Post-lab S35. Starter images slide deck |
Facilitator development session | Facilitators learn how to facilitate Lab C | ~1.5 hours | S27. TA meeting plan |
In lab | |||
Introduction | Introduce the full inquiry lab sequence C, D, and E | 0:00–0:05 |
S26. Lesson Plan S34. Lab C slide deck |
Students ask questions | Students visit each starter image and write questions about each on the poster paper | 0:05–0:25 | S35. Starter images slide deck |
Recap of parallax and inverse-square law | Sort questions |
One facilitator gives presentation that is recap of parallax and inverse-square law. Meanwhile, other facilitator reads and sorts the questions that students wrote on the poster paper. |
0:25–0:40 | S34. Lab C slide deck |
Students choose questions | Students choose questions and form groups | 0:40–0:55 | |
Mini lecture | Mini lecture on taking big questions to investigable questions | 0:55–1:00 | S34. Lab C slide deck |
Start investigation |
Students start on investigations After about ~15 minutes, they share ideas with another group. |
1:00–1:30 | |
Write notes | Hand out worksheets. Students take notes on today’s work. | 1:30–1:40 | S29. In-lab worksheet |
Wrap-up | Congratulate students on their hard work, remind students to work on post-lab assignment | 1:40–1:45 | S30. Post-lab assignment |
After lab | |||
Grading |
Grade In-lab worksheets Grade Post-labs Post pre-lab answers (optional) |
Depends on number of students |
S31. Pre-lab Answer key S32. In-lab worksheet Rubric S33. Post-lab Rubric |
Lab D: Investigations
In Lab D (S36), students carry out their investigations in groups, focusing on measuring the distance to their chosen object. Most work takes place on pieces of poster paper (or whiteboards), so that all group members can contribute and see. Facilitators encourage students to write ideas and sketch diagrams. Each investigation is different, but all involve figuring out a chain of reasoning that takes them to needing a distance measurement (e.g., Figure 5), and then figuring out how to make the measurement, and with what data. Students don’t generally take data in this lab, but rather come up with a method, and facilitators give them the data they need once they’ve figured out the method (e.g., Figure 6). We provide separate facilitation guides and simulated data for three types of investigations: Parallax Type 1 (Solar System: S58–S60), Parallax Type 2 (nearby stars: S61–S70), and ISL (Kepler stars: S71–S75). We describe one possible investigation path here (see S59 and S60).
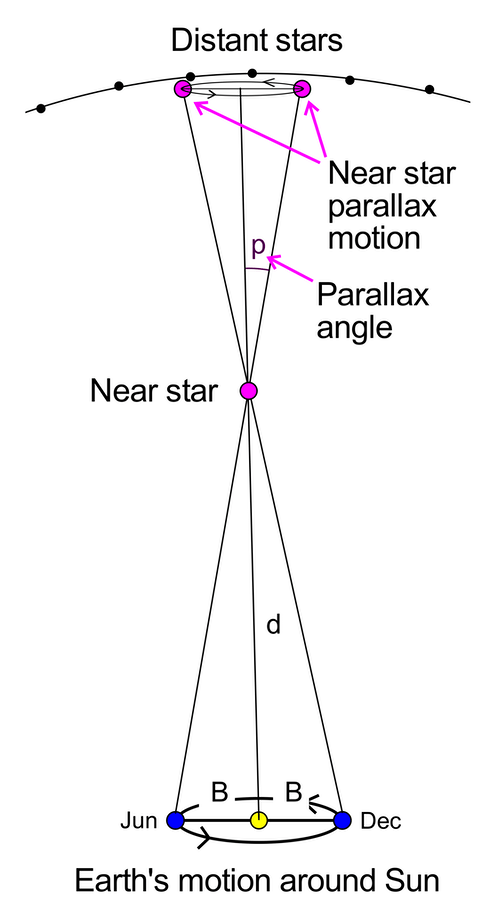
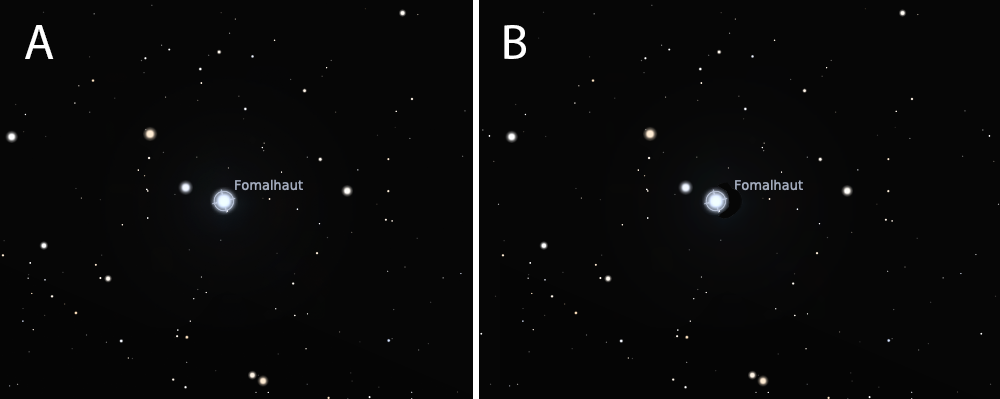
Students select the question, “What are those dark patches on the Moon?” and form a group. Next they brainstorm sub-questions that can help answer their primary question, such as, “What are the physical characteristics of those patches?” They list characteristics of interest including composition, size, and age. Facilitators encourage the group to start by focusing on size, because we have data and materials in the lab to work on answering that sub-question. Through brainstorming and discussions, students realize that they can measure the physical size of dark patches if they can compare them with the physical size of the Moon. Then they recognize that they can measure the physical size of the Moon by comparing the angular size of the Moon with the distance to the Moon. They brainstorm and sketch how to measure the Moon’s angular size (which they can estimate easily with their fingers outside if the Moon is visible!—or otherwise the facilitators can tell them the result once they’ve figured out the method), and then turn their attention to how they could measure the distance to the Moon.
Remembering Lab A, students come up with the idea of using parallax to measure the distance to the Moon. They generally decide to measure the apparent location in the sky of the Moon from widely separated locations on Earth, then recognize that they need a reference object to make such tiny difference measurements. At this point, the facilitator tells the group about a type of astronomical event called a lunar occultation, where the Moon passes between Earth and a bright star. We give students a drawing (as data) of a lunar occultation of the star Regulus, with tracks showing the apparent path of Regulus entering and exiting occultation, viewed from a dozen different cities in the Western hemisphere. Students sketch a diagram and use trigonometry to create an equation connecting the physical separation of two cities, the angular separation of the star’s apparent track behind the Moon as viewed from those two cities, and the distance to the Moon. Then they calculate the distance to the Moon.
The investigation paths are designed so that there are different layers of complexity for students to explore, depending on how quickly they progress. For example, in this investigation path, students can figure out the distance between two cities using trigonometry with their latitude and longitude, or they can estimate the distance (e.g., 2000 km). They can use their distance result to calculate the size of the Moon and size of the dark patches, using the original image. In the investigation paths for planet distances, students can measure the distance to Venus and use that to measure the distance to the Sun and to other planets, or they can just stop when they have measured the distance to Venus. In some cases, students can repeat their distance measurement using a different technique or set of data. With additional guidance towards the end of the lab period, the facilitator can ensure that every group completes a distance measurement. This allows all students to feel a sense of accomplishment, reach the content learning goal, and present their result in Lab E. The in-lab / post-lab assignment (S38) helps students review what data they used in their investigation and how they used it.
Note that investigation paths for more distant objects rely on measurements of closer distances; e.g., to use stellar parallax, we need to know the distance from the Earth to the Sun, which is determined by parallax using the size of the Earth as baseline. When a student group discovers that they need this type of information, we point them to another group of students who are making the type of measurement that they need. So that they can work in parallel, we give the original group the number that they need but remind them that they should check in with the other group at the end of the lab. This highlights the importance of interdependence and scientists working together.
Table 7. Lesson plan timeline for Lab D - Investigations. (S36. Lesson Plan)
Activity | Description | Timing | Associated Documents |
---|---|---|---|
Before lab | |||
Lab preparation |
Gather materials Print In-lab / Post-lab worksheets Print data sheets [No pre-lab this week] Post Presentations assignment on LMS |
~30 min |
S36. Lesson Plan Data sheets: Docs S58-S75 S38. In-lab / Post-lab worksheet S39. Presentations Assignment (in preparation for Lab E) |
Facilitator development session | Facilitators learn how to facilitate Lab D | ~1.5 hours | S37. TA meeting plan |
In lab | |||
Introduction | Recap last week; bring students to study size, brightness or distance | 0:00–0:05 |
S36. Lesson Plan S41. Lab D slide deck |
Students work on investigations |
Students work on their investigations in their groups, with support from facilitators as appropriate Investigation milestones with suggested times are given in the Lesson Plan |
0:05–1:30 |
Docs S58 - S75: Facilitation guides and data for:
|
Students work on In-lab / Post-lab worksheet |
Describe presentation assignment Students work on In-lab / Post-lab worksheet |
1:30–1:40 | S38. In-lab / Post-lab worksheet |
Wrap-up | Congratulate students on their hard work, recap what they did, remind students to work on presentation | 1:40–1:45 | S39. Presentations assignment (in preparation for Lab E) |
After lab | |||
Grading |
Grade In-lab / Post-lab worksheets [We do not recommend posting answers to this assignment] |
Depends on number of students | S40. In-lab / Post-lab worksheet Answer key and rubric |
Lab E: Presentations and synthesis
Students present their results in Lab E (S42), the final lab of the sequence. Groups are asked to spend 1–2 hours preparing a short (5–7 minute-long) presentation using a few slides, a whiteboard, or a poster. The presentation should address the following prompts (S39):
-
What was your question? How does distance help you to characterize your object and answer your question?
-
Describe how you measured the distance to your object. What data and observations did you need, and how did you use them?
-
Describe any assumptions or limitations of your distance measurement technique (e.g., Do you think it works if the object is very close or very far away? Why or why not?).
-
What were your conclusions from your investigation?
We order the presentations from the nearest to the most distant object. Students in the audience write brief structured notes on each other’s presentations (S44). Audience members and facilitators may ask a few questions after each presentation. After the presentations, the facilitator gives a short presentation (S51) reviewing all distance measurement techniques, highlighting how each further distance relies on a closer distance measurement (“the cosmic distance ladder”), highlighting the scientific practices students engaged in, and commending everyone for their work during the lab sequence.
The final piece of the lab sequence is a take-home lab report (S45, S49, S50) that students work on individually to synthesize their learning. The lab report should include an explanation of what question the student investigated, why they were interested in that question, how they broke it down into smaller sub-questions, how those sub-questions help to answer the big question, how they made their distance measurement including diagrams and equations, assumptions and limitations in their measurement technique, and reflections on how their group worked together and what they learned about being a scientist. Students also answer the same reflection questions presented after Labs A and B (for Labs C, D, and E as a whole; S55).
Table 8. Lesson plan timeline for Lab E - Presentations and synthesis. (S42. Lesson Plan)
Activity | Description | Timing | Associated Documents |
---|---|---|---|
Before lab | |||
Lab preparation |
Print In-lab worksheets Post Lab Report assignment on LMS Post Post-lab survey questions on LMS |
~15 min |
S44. In-lab worksheet - Audience notes S45. Lab Report Assignment S55. Questions for students after labs A, B, E |
Facilitator development session | Facilitators learn how to facilitate Lab E | ~1.5 hours | S43. TA meeting plan |
In lab | |||
Introduction | Welcome students, determine order of presentations | 0:00–0:05 |
S42. Lesson Plan S51. Lab E slide deck |
Student presentations |
Students present their investigations to their classmates. Audience members and facilitators ask questions |
0:05–1:30 |
S44. In-lab worksheet - Audience notes S46. Presentation Scoring sheet S47. Presentation Scoring - Notes for Facilitators |
Synthesis and wrap-up | Congratulate students on their hard work, review what we have learned about distance measurement and scientific practices, give overview of lab report assignment | 1:30–1:45 |
S51. Lab E slide deck S45. Lab Report Assignment |
After lab | |||
Immediately after lab | Go back through your notes on each presentation in case you want to revise or add anything. Do this right after lab so you don’t forget. | Depends on number of students |
S46. Presentation Scoring sheet S47. Presentation Scoring - Notes for Facilitators |
Grading |
Grade In-lab worksheets (Audience notes) Grade lab reports |
Depends on number of students |
S48. In-lab worksheet Rubric S49. Goals for Lab Report S50. Lab Report - Rubric |
Inquiry facilitation and facilitator development for teaching assistants
The effectiveness of an inquiry lab depends on both the curriculum and how the curriculum is facilitated. Facilitating inquiry is quite different from other teaching approaches, including other interactive labs. The overall aim is to support students as they work together in a team to ask their own questions and figure out their own discoveries. Stefanou et al. (37) describe a similar aim as supporting students’ cognitive autonomy, “empowering students to develop self-reliance of thinking.” The Exploratorium Institute for Inquiry (38) gives an overview of the facilitation philosophy; see also (39). Facilitators listen, use words and body language, collaborate, and reflect to support students’ learning and equitable group dynamics. Important features of how we aim to facilitate include:
-
Attitude towards students:
-
Explicitly assuming that students will be and are interested and engaged.
-
Setting challenging positive expectations that students can succeed in the lab and in science in general. This includes giving encouragement and reiterating the sense that “you can do it.”
-
Emphasizing effort and thinking process rather than answers; thanking students for their hard work.
-
-
Finding out what students are doing and thinking, and using what we find out to support students along their individual paths of learning while maintaining their ownership of their discoveries.
-
Watching and listening more often than speaking to the group, to encourage the group to develop their own ideas without looking to an “expert.”
-
Being respectful: asking permission before touching materials, drawing on their poster paper, etc.
-
Timing questions and suggestions carefully to build on students’ current level of understanding, e.g., when students have been stuck for a while or the next step would be too difficult or time-consuming to figure out on their own.
-
Holding back from offering suggestions. This recognizes that telling students answers can often lead to them trying to understand what they were told rather than trying to understand the phenomenon.
-
Giving stronger suggestions towards the end of the lab period, to help all students reach the learning goals.
-
-
Supporting equitable group dynamics. For example, encouraging quieter members to speak, sometimes asking more dominant members to hold back, making sure everyone has the opportunity to share ideas and write or draw on the poster paper, making sure everyone’s ideas are engaged with and everyone understands when the group moves forward.
-
Checking in often with fellow facilitators, to learn how other groups are progressing, support each other with new or challenging situations, etc.
-
Writing reflections on our facilitation experience following the labs.
Since inquiry facilitation was unfamiliar to most of our TAs, we devoted significant energy towards facilitator development. We began by recruiting TAs motivated in learning to facilitate inquiry, via an application process. The first stage of development (S52) was a special session in which TAs engaged in (an abbreviated version of) the lab sequence pretending to be students, then reflected on their experience, similar to in the ISEE Professional Development Program (39). This is to give TAs the feeling of making discoveries in this type of lab, so they know what they are aiming to help their students experience. TAs also read short readings about inquiry and facilitation, and we discussed them as a group. Later in the term, we held TA sessions for each individual lab, tailored to lab specifics (S2, S14, S27, S37, S43). The other key feature of our TA development was a mentorship paradigm (similar to paired teaching; see [40]): Each week, the first lab session was facilitated by LS and DG (STLF and Inquiry TA); subsequent lab sessions that week were facilitated by one of LS or DG plus another TA. In that way, LS mentored DG, then each of us mentored the other TAs in our sections. Bohrer et al. (41) also highlight modeling and mentorship as important aspects of TA development for inquiry facilitation. After each lab, we encouraged TAs to write observations of the labs and reflections on their facilitation experience (S53), and we gathered feedback from TAs after the special development session and at the end of the term, to inform future TA development and future implementation of the labs. We provide our TA development documents as part of the set of course materials.
At the end of the term, one TA summarized that they had learned to make space for students to explore their questions in their own way: that there are learning goals, but students may create unique paths for reaching those goals.
Teaching Discussion
Evaluation of student learning
Overview
As we were introducing inquiry labs to this course for the first time, we wanted to take a scholarly approach to see whether the lab transformation was worthwhile and to suggest future improvements. We examined the labs’ impact from several perspectives, each connected to anticipated outcomes: students’ perceived benefit of the labs within the larger course, their developing attitudes towards the discipline and self-identity as a scientist, their emotional response to the labs, and a performance-based assessment to measure learning of astronomical concepts. We also considered students’ open responses to reflection questions after three of the labs.
Assessments in a typical implementation of this lab sequence would include the pre- and post-lab assignments, group presentation, lab report, and a few questions related to distance measurement on the midterm and final exams—but not all the types of evaluation that we are including in this section, as these were developed specifically to demonstrate the effectiveness of this approach by authors LS and DG.
The data collected here was part of a course/lab improvement project, with information being used to improve the course in subsequent iterations; in our jurisdiction, this type of activity is not subject to institutional ethical review under Article 2.5 of the Tri-Council Policy Statement. We provide summaries and some interpretations of collected data but no direct quotations or artifacts from individual students.
Helpfulness of labs as a course component
In 2016 (before we transformed the labs) and 2017 (first year of inquiry labs), students completed a survey at the end of the course about many aspects of the course, including how helpful they found different course components, both in-class and out-of-class (i.e., during or outside lecture). We present the results for out-of-class course components for both years, highlighting the results for the labs (Figure 7). In 2016, 28% of students found the labs to be not at all helpful or slightly helpful, while 32% found the labs very helpful or extremely helpful. In 2017, only 11% of students found the labs to be not at all helpful or slightly helpful, while 60% found the labs very helpful or extremely helpful. The labs also increased in helpfulness relative to other course components. Thus, we can conclude that the lab transformation led many more students to rate the labs a helpful part of the course.
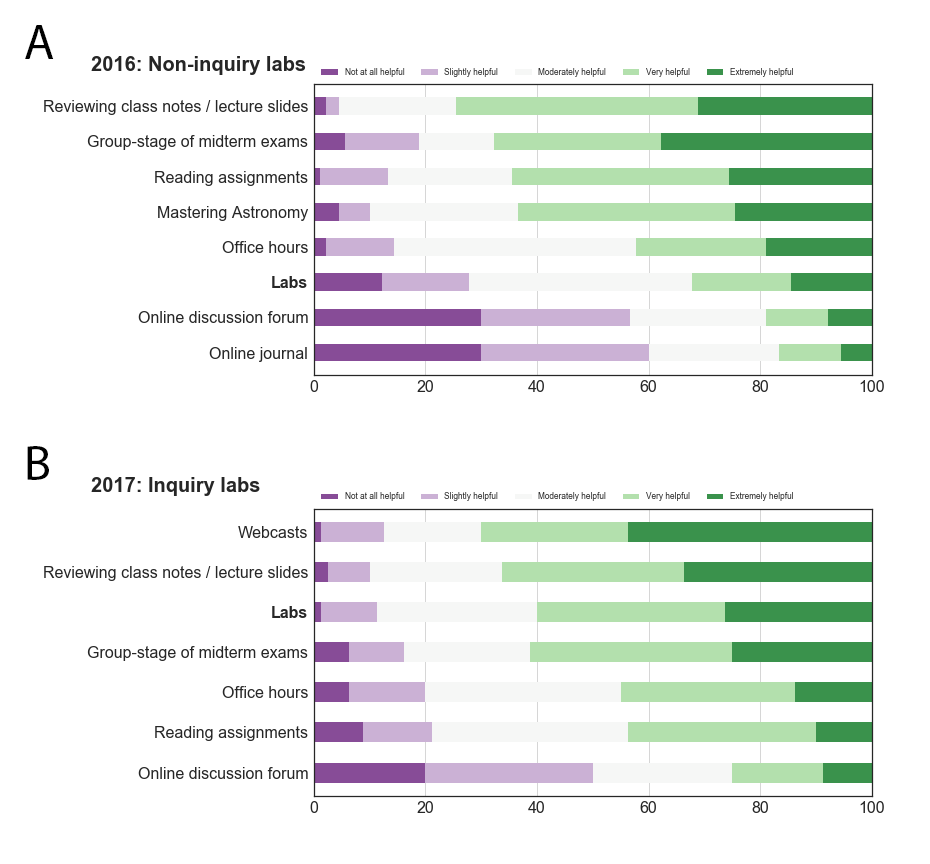
Students’ feelings about their experiences in the labs
Students completed a short survey about their experience in the lab after completing Labs A, B, and E (parallax warm-up, ISL warm-up, and after the full inquiry sequence; S55). Of the six feelings surveyed, three were positive (interested / engaged, confident, proud), and three were negative (anxious, frustrated, confused). In Figure 8, we show all students’ responses (labelled with N on the plots); for only matched student responses, the shapes look similar. In either case (non-matched or matched), the positive emotions are more prominent than the negative emotions, for each of the three labs.
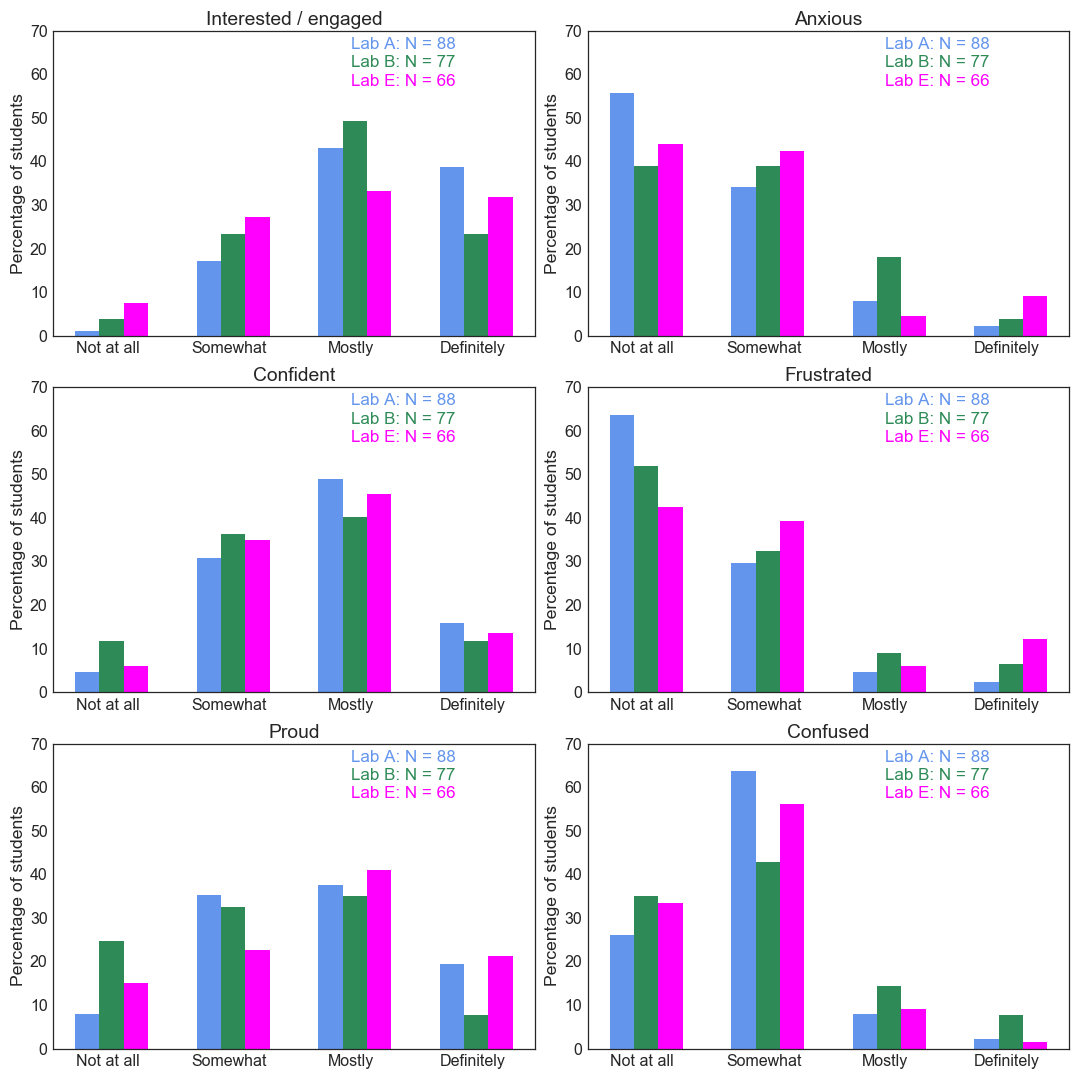
Distance-concept questions (pre/post course)
At the beginning and end of the course, we gave students the Astronomy Diagnostic Test 2.0 (ADT; [42]; available here). As the learning of distance concepts was a focus of the inquiry lab sequence and because few of the ADT questions are related to measuring distances, we also wrote three questions specifically to probe the concepts / phenomenology addressed in the inquiry lab sequence, one of which (Question #22) is adapted from Question #6 in the Size, Scale and Structure Concept Inventory v2.0 (S3CI; [43]; shared by E. F. Ladd, personal communication 2017; not currently publicly available, to our knowledge). Here we focus on the five distance-related questions: #12 and #15 from the ADT, and the three questions we created/adapted (Table 9). The full text of the questions is available in S57. We also note that although these questions were most addressed in the lab, they were also addressed in the lecture component of the course.
Table 9. Percentages of UBC students who answered each distance-concept question correctly, at the start (pre) and end (post) of the course and the Cohen’s d effect size (standardized post – pre difference) for each question with 95% confidence interval, for the N = 69 students who answered both times (i.e., matched responses). Question numbers are labelled with whether the topic is parallax or ISL. Rows in bold had a significant positive effect size.
Question Number | Percentage Correct, pre | Percentage Correct, post |
Cohen’s d Effect Size |
---|---|---|---|
#12* (parallax) | 67% | 68% | +0.03 [-0.21, +0.27] |
#15* (ISL) | 52% | 75% | +0.49 [+0.24, +0.75] |
#22† (ISL) | 83% | 77% | -0.14 [-0.38, +0.10] |
#23‡ (parallax) | 55% | 81% | +0.58 [+0.32, +0.84] |
#24‡ (parallax) | 28% | 39% | +0.25 [0.0, +0.49] |
*Questions #12 and #15 in the ADT 2.0 (41).
†Question adapted from Question #6 in the Size, Scale and Structure Concept Inventory v2.0 (S3CI: [43]); shared by E. F. Ladd, personal communication 2017).
‡Questions created by the authors.
We follow Nissen et al. (44), who recommend using Cohen’s d effect size in analysis of concept inventory scores rather than normalized gain. The Pre average percentage correct on this set of questions is 57% with a 95% confidence interval of [52%, 62%]. The Post average percentage correct on this set of questions is 68% with a 95% confidence interval of [63%, 73%]. We calculated an effect size (Cohen’s d) between post and pre average percentages correct of 0.23, which is a positive shift in line with typical effect sizes for educational interventions (45). However, when we calculated the Cronbach alpha for pre and post scores on this set of five questions, each was low (0.48 and 0.53, respectively), suggesting that the questions may not probe students’ understanding of a single concept. Alternatively, the Cronbach alpha may be low simply because of the small number of questions in the survey (46). We briefly discuss the questions individually in S57.
While these questions may need further refinement, they could inform future development or revision of a concept inventory about distance measurement (e.g., the S3CI). Overall, our results suggest that the inquiry labs supported students’ learning of the concepts of parallax and ISL, and how these relate to measuring distances in the Universe.
In this paper, we focus on assessing students’ conceptual understanding via the ADT 2.0 and S3CI distance measurement questions, because they are standardized and relatively easy to analyze and share results. However, a typical implementation of this lab sequence would primarily assess students’ conceptual understanding via the post-lab assignments, group presentation, and lab report (all documents given in the Supporting Materials with answer keys and rubrics), and questions on midterm and final exams (not included). These assessments can provide rich information to instructors on how well students are learning about concepts in astronomical distance measurement. While there is not yet much research on student learning of astronomical distance measurement, (43, 47, 48) are a few articles that touch on this topic. Research on student understanding of astronomical distance scales—though not distance measurement—includes (49) and (50).
CLASS
Students completed the Colorado Learning Attitudes about Science Survey – Physics (CLASS; [25]; available here) at the beginning and end of the course in 2015 (before course transformation), 2016 (lecture transformed, labs not transformed), and 2017 (lecture transformed, inquiry labs introduced). Each year of the course had a consistent negative shift (calculated with the PhysPort Data Explorer), shown in Figure 9. This type of shift, while disheartening, is unfortunately common even in highly interactive classrooms (27).
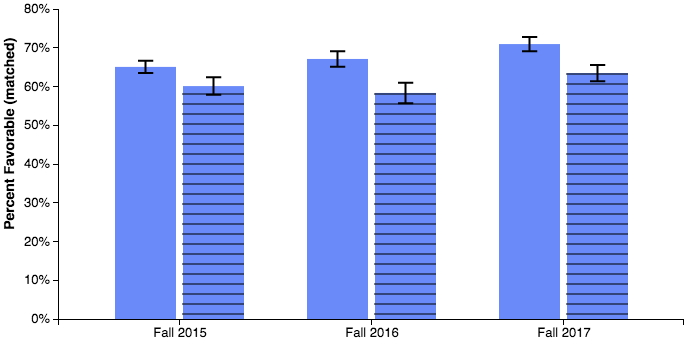
Although the CLASS offers one view of our student populations, we find these results have limited value for understanding how the inquiry lab sequence impacts students’ learning. First, the survey refers to physics not astronomy (there is no CLASS-astronomy): students likely see these as separate fields and may be answering the questions with their physics course(s) in mind. Second, students’ responses to the CLASS are influenced by the course as a whole, not specifically the inquiry labs. (We note that the E-CLASS [51], a lab-specific attitudinal survey, does not focus on the types of attitudes we focused on in our inquiry lab sequence.) The inquiry lab sequence comprised only about half of the labs in the course, and the labs in total were only about one-third of the course hours. So even if the inquiry labs did help develop students’ attitudes and beliefs about science, more of the rest of the course may need to be oriented towards scientific model-building (27) for CLASS results to change appreciably.
Self-identity as a scientist
One aim of the lab sequence was to help students to grow their self-identity as scientists. At the beginning and end of the term, we gave students a modified version of a subset of questions from the Sustainability and Gender in Engineering (SaGE) Project (33, 52, 53), accompanying the CLASS. This subset was developed to learn about first-year undergraduate students’ physics identity (and is available in Lock et al. [33]’s Table II); we modified their questions to refer to “science” rather than “physics.” Students rated their agreement to prompts such as “I understand concepts I have studied in science,” and “My parents / relatives / friends see me as a science person.” In the SaGE project, students took the survey only once, to correlate with other factors, not pre/post to measure the impact of an educational intervention.
Following Lock et al.’s analysis (33), we converted the Likert scale (Strongly disagree to Strongly agree) to integers 0–4. We calculated the mean survey response value across all students for pre and post [with 95% confidence intervals] for the set of questions as a whole, and also for the subsets of questions corresponding to the three self-identity constructs identified by Lock et al. (33); results are shown in Table 10. We calculated the effect size comparing post and pre scores using Cohen’s d. There may be a small drop for each of the constructs. Nevertheless, the values for each start and remain quite high (close to “Agree”).
Table 10. UBC students’ (N = 77) mean response values for the survey about self-identity as a scientist, at the beginning (pre) and end (post) of the semester [with 95% confidence intervals], calculated from a Likert scale converted to 0–4 (Strongly disagree to Strongly agree). Cohen’s d is the standardized mean difference post-pre. Results are shown for the set of all self-identity questions, and also for subsets of questions corresponding to the three self-identity constructs identified by Lock et al. (35).
All Self-Identity Questions | Performance / Competence | Recognition | Interest | |
---|---|---|---|---|
Pre | 3.07 [3.01, 3.13] | 3.05 [2.97, 3.12] | 2.79 [2.64, 2.95] | 3.42 [3.31, 3.54] |
Post | 2.95 [2.89, 3.00] | 2.89 [2.82, 2.96] | 2.72 [2.57, 2.87] | 3.33 [3.23, 3.43] |
Cohen’s d | -0.15 [-0.38, +0.08] | -0.20 [-0.42, +0.03] | -0.07 [-0.30, +0.15] | -0.13 [-0.36, +0.09] |
Further, like the CLASS, this survey relates to the course as a whole and not specifically to the influence of the inquiry labs. (For example, “I can do well on exams in science” does not connect directly to a student’s experience in the labs.) In addition, some of the questions measure students’ changing confidence and relation to other students and instructors, as they move through their first semester in university. For minoritized students, prompts such as “My teachers see me as a science person” may be more about biases and stereotypes of the teachers than about the self-identity of students themselves. For a future survey, we might like to give prompts more like, “I feel like a scientist,” “I can envision myself as a scientist,” and “I can see my path to becoming a scientist.” We note that Godwin et al.’s SaGE survey (53) did include the statement “I see myself as a [physics] person.” We did not include this statement because it was not mentioned in Lock et al. (52), presumably because they do not use it as part of a separate construct.
Students’ reflections on their learning
As part of helping students reflect on their learning and approach to the inquiry labs, we gave students open-ended reflection questions (graded for completion) after three of the labs (A, B and E; those after Lab E asked students to reflect on their experience in Labs C, D, and E as a whole). The prompts (S55) were (variations of):
-
“What did you learn in this week’s lab about conducting a scientific investigation?” (Labs A, B, and E)
-
“What went well in your approach to today’s lab? How might you approach the future inquiry labs differently than you approached today’s lab?” (Labs A, B, and E)
-
“Think of other lab classes you’ve taken or are taking, either at university, or in high school. What did you get out of this week’s lab that was different from other lab classes you’ve taken or are taking (if anything)? What advantages and/or disadvantages do you see for learning this way?” (Labs A and B)
Although these were not given for grading purposes, students’ responses to these questions give us richer, more detailed insight into their experience in the labs than their responses to the surveys described above. Here, we highlight a few themes that stand out and may suggest avenues for future investigation.
Theme 1. Students appreciated having freedom to come up with their own approach in the lab.
In response to Question #3 following Lab A, fully half (23/46) of the students mentioned appreciating this freedom. Students expressed a variety of reasons for appreciating this freedom, including being helpful for learning concepts and problem-solving; being more interesting, motivating and rewarding; encouraging creativity, and feeling more like real scientific research. The tone of many students’ responses was enthusiastic. This theme seems strongly connected to cognitive autonomy (e.g., [37]), in which students feel motivated by having the independence to originate their own ideas and to think and make meaningful decisions about the subject in their own way.
A few students also noted disadvantages of this freedom along with such advantages, e.g., that learning this way is more time-consuming, and that (without TAs checking in) students can be on the wrong track without realizing it. It can also raise anxiety not to know exactly what is expected of you when you enter the lab.
Theme 2. (a) Students discovered that real-life science is challenging. Two ways to help succeed through the challenge are (b) breaking big science questions into smaller questions, and (c) working together in a team.
After Lab E, 7 out of 43 students (16%) expressed discovering that scientific investigations are challenging. While this could sound like an undesirable outcome, we suggest that: (i) it’s an adjustment to a realistic view of science—sooner or later, all science students will discover that investigations are challenging—and (ii) it can be positive if learned in combination with strategies to address that challenge.
A primary strategy that students mentioned was breaking down bigger, more challenging questions into smaller questions, a scientific practice that we emphasized in Lab C. In response to Question #3 after Lab E, 18 out of 43 students (42%) mentioned learning this practice, and some connected this to their own ability to solve scientific problems. Several students noted that their experience in the labs was similar to how scientists work. These results show students building their self-efficacy in conducting investigations.
The second strategy for addressing challenges in science was working together in a team; in response to Question #2 over Labs A, B, and E combined, this was mentioned in 55 out of 150 student responses (37%). Students mentioned the importance and benefits of teamwork for their success in the labs; e.g., each member sharing their different questions and ideas to come to a solution together, and a feeling of camaraderie in working together through a challenging task.
In this second theme, we see an intertwining of students’ learning an idea about the nature of science (investigations are challenging) while developing their self-efficacy for conducting investigations. This intertwining resonates with Quan & Elby (54)’s results for undergraduate students’ developing their self-efficacy for conducting scientific research, intertwined with learning that people at all levels can contribute to research (an idea about the nature of science).
Inquiry activity at the Pan-African School for Emerging Astronomers (PASEA)
This inquiry sequence was originally created for the Pan-African School for Emerging Astronomers (PASEA; formerly the West African International Summer School for Young Astronomers—WAISSYA), through the Institute for Scientist and Engineer Educators Professional Development Program (ISEE PDP) at the University of California, Santa Cruz. PASEA is a week-long short course in astronomy for African university students; see (11). We have taught the inquiry activity in the undergraduate stream of each edition of the program (2013, 2015, 2017, and 2019, in Nigeria and Ghana), and again in Zambia 2022 (postponed from 2021 due to the COVID-19 pandemic) just before this article went to press.
PASEA students are undergraduates interested in astronomy, typically in their third year; many are physics or engineering majors (a few are astronomy majors, though astronomy degree programs are rare in Africa). Our students are highly motivated; they have applied and invested significant time and energy to participate in this voluntary program. We typically have about 60 students and six inquiry facilitators.
While the learning goals and teaching activities are similar between UBC and PASEA, the formats are different; we compare them in Table 11. The concluding activity at PASEA is a facilitated jigsaw discussion (see this site about the jigsaw technique): these discussion groups comprise one member from each lab investigation group who informally describes their question and results to their classmates, supported by a facilitator. We compare students’ engagement with the different formats later in this subsection.
Table 11. Comparison of formats between the inquiry labs at UBC and at PASEA. Both formats have similar learning goals and teaching activities. For more detail about the types of astronomical objects, see Table 4.
Lesson Structure | UBC | PASEA |
---|---|---|
Schedule in class | Five weeks = 10 hours | Two days (half, full, half) = 12 hours |
Work required outside of class | Pre-lab and post-lab homework assignments, preparation of presentation and lab report | None |
Graded assessments? | Yes | No |
Types of astronomical objects | Solar System and nearby stars only | Solar system, nearby stars, galaxies, supernovae |
Concluding activity | Group presentations and lab report | Jigsaw discussion |
We support new instructors to learn inquiry facilitation by first engaging them in a mock version of the activity as students, then partnering each new instructor with an experienced facilitator to discuss specific facilitation strategies, ask questions, debrief, etc. while facilitating the student investigations (Figure 10). Because the PASEA curriculum includes four types of astronomical objects corresponding to four different investigation paths, facilitators often specialize in one path.
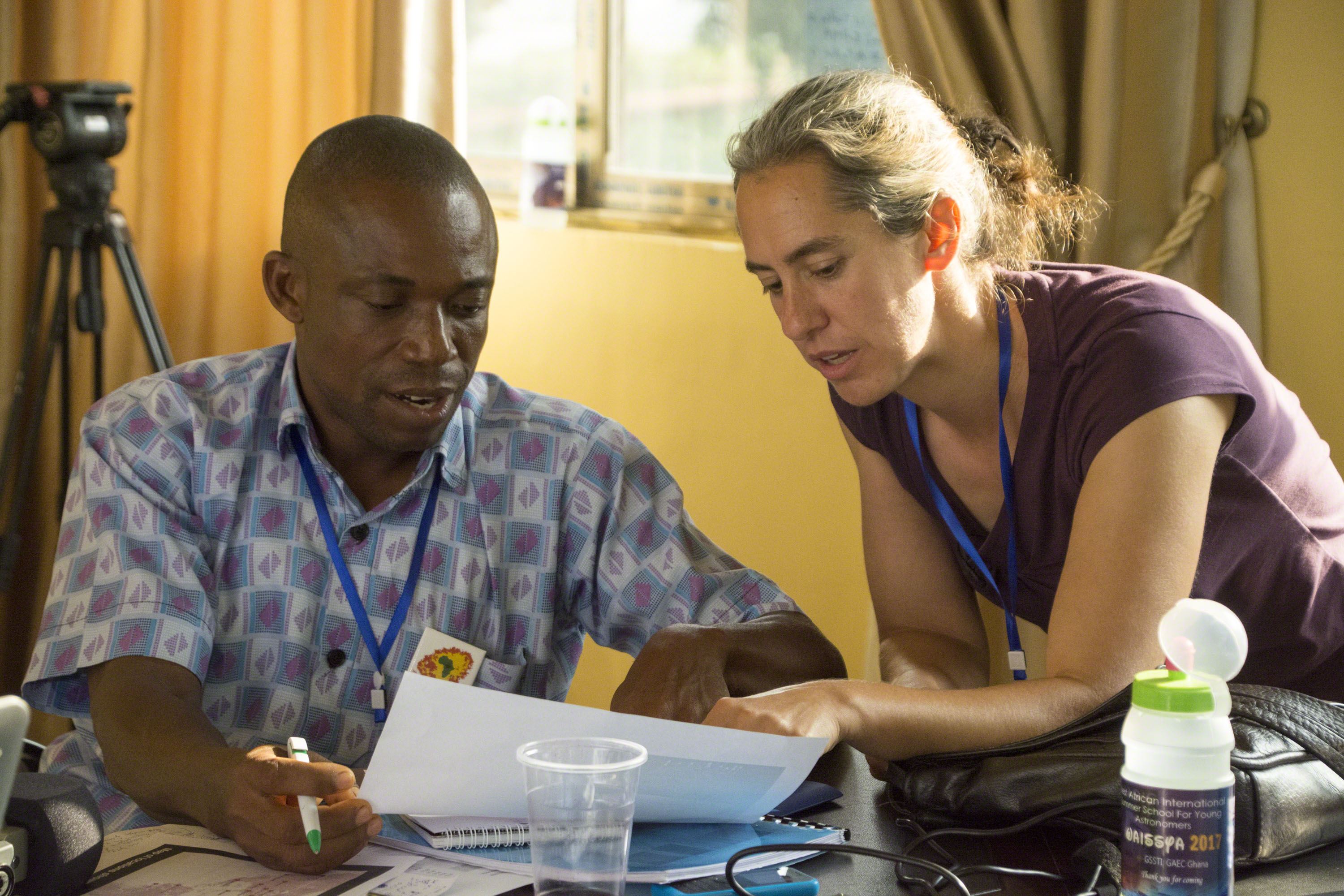
At each edition of PASEA we have experimented with and revised the inquiry curriculum at PASEA based on past experiences. For example, in the past we have asked students to create a scale model of the Universe together based on their investigation results and given students a set of problems to solve on their own as a post assessment. We developed the inquiry warm-up activities about parallax and ISL in response to seeing in the first year that students needed to explore these concepts separately before engaging in the full inquiry. We brought our “hint sheets” from UBC back to PASEA in 2019. Teaching inquiry in two very different contexts has been fruitful for trying and exchanging teaching ideas back and forth.
The different formats and contexts at UBC and PASEA led to differences in how students engaged with the inquiry. LS (the only instructor in common between UBC and PASEA) had the impression that UBC students tended to create more mathematically precise parallax-related methods (Lab A) and to give more sophisticated explanations of their astronomical distance measurements (Lab D/E), relative to PASEA students. This is likely because UBC students, in contrast to PASEA students, spent required time on the inquiry activity outside of class reviewing relevant concepts (e.g., trigonometry) in the pre-lab assignments and synthesizing results for their presentations; it may also be because UBC students knew that their work would be graded. Meanwhile, PASEA students tended to engage in the inquiry with more curiosity, motivation, and passion than UBC students. At PASEA 2019, most groups stayed working on their investigations well into the evening after the allotted time was over, because they wanted to figure out the answers to their questions; cheers of accomplishment and joy erupted around the room as each team reached success. Lack of graded assessments at PASEA helped maintain the feeling that everyone was there to learn for their own innate curiosity and intellectual satisfaction, not to meet outside requirements—an illustration of the idea that autonomy is a key component of intrinsic motivation (55). Inquiry was a new way of learning for most UBC students and essentially all PASEA students. PASEA students particularly embraced it, perhaps because PASEA is a special opportunity for which they volunteered and were selected. The stark difference from usual teaching approaches in their experience likely contributed to students feeling excited and motivated.
We gave students the CLASS in 2015, 2017, and 2019, at the beginning and end of the week-long program. We share results in Figure 11, with several caveats: (i) similar to at UBC, the CLASS reflects students’ responses to the program as a whole rather than the inquiry activity in particular; (ii) the CLASS has not been validated with African university students, whose educational system, culture and often English usage are quite different from those of the U.S.-based students for whom the CLASS was created and validated; and (iii) we had some challenges matching students between pre- and post-surveys, and with students skipping questions, so unfortunately many of our PASEA students’ CLASS responses are not included in our analysis. Nevertheless, we found that our students’ CLASS scores have shown positive shifts with growing effect size each year, up to d = 1.31 (a very large effect size) in 2019. This strong positive shift is very rare, as described earlier (27).
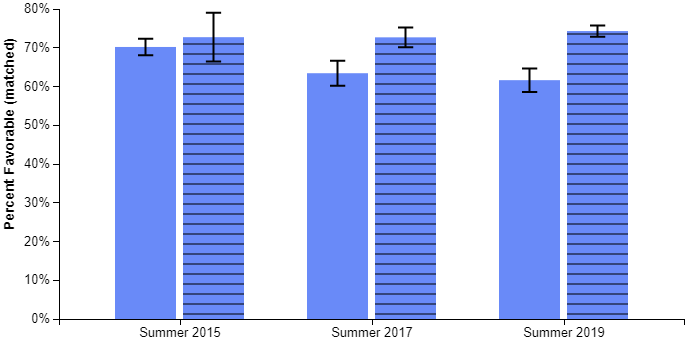
We note that PASEA 2017 and 2019 students’ CLASS scores showed positive shifts in each of the eight CLASS attitude categories (e.g., “personal interest,” “problem solving sophistication”) identified by (25), while UBC 2017 students showed small negative shifts in each category. The different CLASS results for PASEA relative to UBC may be partly due to the different formats and contexts just described, and several other causes. The entire PASEA program (even the non-inquiry components) is an intense experience framed towards building students’ scientific thinking, interest, and self-efficacy, while UBC students’ programs typically have more varied and less coherently framed goals across multiple science classes and multiple components (e.g., traditional as well as inquiry labs, lectures with varied goals), and are more drawn out in time (across the semester). Another factor may be that UBC students’ average Pre scores started higher than those of PASEA students.
In our wrap-up each year, students orally share reflections on the inquiry activity; while these are mostly not recorded, each year the instructors have felt the students’ heartfelt motivation, pride, growing self-identity as a scientist, and the sense that participation in the inquiry activity has been transformative. In one written reflection, PASEA students (like UBC students) also connected their developing self-efficacy with learning to think like a scientist.
Discussion
We have presented a sequence of inquiry-based labs about distances in the Universe, with implementations in two different contexts: the lab component of an introductory astronomy course in North America, and as the focal activity of an astronomy summer school program for university students in West Africa. We consider the inquiry lab sequence to be a success. At UBC, most students found the inquiry labs to be very helpful (in particular, relative to the non-inquiry lab sequence of prior years) and had positive feelings after the labs. Students had significant learning gains on several conceptual questions related to parallax and ISL. Many students appreciated the freedom to think and explore problems in their own way and grew their self-efficacy to conduct scientific investigations. At PASEA, students’ scientific attitudes became more sophisticated, and many found the inquiry activity to be transformative in their development as scientists. In parallel, learning to facilitate inquiry helped UBC TAs and PASEA instructors learn new ways to teach.
Although all students were science majors, we suggest that this lab sequence could be successful with non-science majors as well. Many non-science majors are inspired by the Universe, as science majors are. Developing the scientific practices of asking questions, breaking down bigger questions into smaller questions, designing investigations, sketching diagrams, synthesizing and presenting findings, and working in a group are all highly relevant and accessible to non-science majors. Discovering that scientists measure astronomical distances in ways that have everyday analogues is perhaps even more powerful for non-science majors than science majors. Nonetheless, we expect that these labs may work best for students who have some facility with basic algebra and trigonometry, so that they can design and carry out their own distance calculations. Successfully making these calculations concretizes and sharpens students’ understanding of the distance measurement techniques. It also contributes to students’ sense of pride and self-identity as scientists: students recognize that they have made their own distance measurement as scientists do, which is a powerful aspect of these labs. Implementing this lab sequence for non-science students in a course with no math would require some substantial modification to find ways to create a similar sense of accomplishment for students without them making their own calculations.
One component of the lab sequence that would benefit from further revision is the hands-on component of Lab B about ISL. Many students come with the unexamined assumption that apparent brightness must decrease as r-1 (instead of r-2)—or if they’ve previously learned the ISL, it is memorized knowledge without deep understanding of why it is true. It is challenging to visualize light rays spreading out over the surface of a growing sphere (i.e., why the ISL applies), and our shadow puppets activity could be more effective in helping students create that three-dimensional visualization in their minds. We’ve tried creating 3D models of nested spheres (though these are hard to construct) and prompting students to draw a 2D projection of the spreading light rays (though this may inadvertently reinforce the idea of r-1 instead of r-2). We find that giving students a light meter to use tends to complicate the issue with measurement error rather than clarifying the reason for the ISL relationship. We encourage instructors to continue experimenting with this lab.
We highlight that students were successfully able to ask their own questions to investigate without having to rely on an authority. This is significant because it is an important scientific practice and piece of the nature of science, relevant far beyond science classes and including for students who do not become scientists. It is further significant because many lab curricula, even those referred to as inquiry (e.g., Physics by Inquiry [22]; ISLE [16]), do not include students asking their own questions to investigate. Slater et al. (20) do build up to this practice but only “allow” students to create their own questions in the final stage of their inquiry sequence; their strategy for teaching this practice is to have students engage repeatedly with investigable questions written by the instructors, and eventually learn to identify and write appropriate questions by having “seen so many.” While we acknowledge the merits of this strategy, we suggest that it may encourage students to try to imitate questions generated by authority (against the nature of science), rather than honoring students’ creativity and sense of agency to ask questions based on their own curiosity and in their own way. Our strategy has students freely brainstorm many questions they are interested in, instructors select a set of investigable questions from this longer list (which does still introduce authority for validating questions), and then students collaboratively brainstorm smaller questions that contribute to understanding their bigger questions. This brainstorming progression worked successfully and built self-efficacy for both North American and West African university students, growing their confidence to solve problems in these labs and in other scientific research as well.
Moreover, many students expressed appreciating thinking deeply about topics they value and feeling challenged. We suggest that students feel motivated by the cognitive autonomy designed into the labs: our inquiry curriculum is designed to be open enough that students can explore astronomical topics they are interested in (because measuring distance underlies many areas of astronomy)—i.e., they value learning about the questions they are investigating—and they have significant freedom to create and feel ownership over their own ways of thinking about their investigations. The two themes we found in students’ written reflections highlight the importance of motivation, autonomy, and self-efficacy (all components of agency; [56]), intertwined with scientific practices and the nature of science, and suggest interesting directions for future study. Further research studies of inquiry-based learning outside North America would be particularly valuable.
In conclusion, we encourage other astronomy instructors to try inquiry—including students asking questions—in their courses, and suggest future study of how inquiry can particularly support students’ motivation, autonomy, and self-efficacy.
Supporting Materials
Table 12A. List of supporting files for Labs A–E. Full file names follow this pattern (e.g.,):
S1. Distance Inquiry - Lab A - Lesson Plan
S28. Distance Inquiry - Lab C - Pre-lab
In the table below, we list the file names dropping the phrase “Distance Inquiry - Lab X” for conciseness. In all files, any image where no image credit is listed was created by the curriculum authors.
Lab Name | Plans | Documents for Students | Answer Keys and Rubrics | Slides |
---|---|---|---|---|
A |
S1. Lesson Plan S2. TA Meeting Plan |
S3. Pre-lab S4. In-lab worksheet S5. In-lab hint sheets S6. In-lab Trig Tip Sheet S7. Post-lab - Worksheet version S8. Post-lab - Version to post on LMS |
S9. Pre-lab Answers S10. In-lab worksheet - Rubric S11. Post-lab Answer key and rubric S12. Post-lab Answer key to share with students |
|
B |
S13. Lesson Plan S14. TA Meeting Plan |
S15. Pre-lab (with answers) S16. Worksheet 1 - Sedna S17. Worksheet 2 - Shadow puppets S18. Worksheet 3 - Hemispheres S19. In-lab Light rays hint sheet S20. In-lab List of constants S21. Post-lab |
S22. Worksheet 1 - Sedna - Answer Key S23. Worksheet 2 - Shadow puppets - Answer Key S24. Worksheet 3 - Hemispheres - Answer Key S25. Post-lab Answer key and rubric |
|
C |
S26. Lesson Plan S27. TA Meeting Plan |
S28. Pre-lab S29. In-lab worksheet S30. Post-lab |
S31. Pre-lab Answer key S32. In-lab worksheet Rubric S33. Post-lab Rubric |
S34. Slide deck S35. Starter images |
D |
S36. Lesson Plan S37. TA Meeting Plan |
S38. In-lab / Post-lab worksheet S39. Presentations Assignment (in preparation for Lab E) |
S40. In-lab / Post-lab worksheet Answer key and rubric | S41. Slide deck |
E |
S42. Lesson Plan S43. TA Meeting Plan |
S44. In-lab worksheet - Audience notes S45. Lab Report Assignment |
S46. Presentation Scoring sheet S47. Presentation Scoring - Notes for Facilitators S48. In-lab worksheet Rubric S49. Goals for Lab Report S50. Lab Report – Rubric |
S51. Slide deck |
Other documents:
- S52. Pre-semester TA Meeting Plan
- S53. Facilitator reflection prompts
- S54. Notes for grading inquiry labs
- S55. Questions for students after labs A, B, E
- S56. Inquiry labs overview - for students
- S57. Distance concept inventory questions and analysis
Table 12B. List of supporting files for Lab D - Data and information for three investigation paths.
Parallax Type 1: Solar System | Parallax Type 2: Nearby stars | ISL: Kepler stars |
---|---|---|
S58. Facilitation guide (short version) S59. Facilitation guide (full version) S60. Description of the simulated data |
S61. Facilitation guide S62. Description of the simulated data Data: S63. 51Peg_Stellarium.png S64. 51Peg_Stellarium_shifted.png S65. Fomalhaut_Stellarium.png S66. Fomalhaut_Stellarium_shifted.png S67. Gliese445_Stellarium.png S68. Gliese445_Stellarium_shifted.png S69. ProximaCen_Stellarium.png S70. ProximaCen_Stellarium_shifted.png |
S71. Facilitation guide S72. Set of template stellar spectra - For students S73. Set of template stellar spectra - Answer key Data: S74. Kepler 20 Spectrum S75. Kepler 20 Light curve |
Acknowledgments
We are grateful for contributions to the development of this inquiry lab sequence by Valerie Murray, bringing her classroom experience of many years as a science teacher in the San Francisco Bay Area and Toronto; comments on the article draft by James Chibueze, Adaeze Ibik, Margaret Ikape, and Bo Mapfumo; contributions to the PASEA CLASS analysis by Sera Rauhut; help with references about inquiry facilitation from Nicholas McConnell; and discussions about UBC assessments with Sarah Perez. We are grateful for support and feedback on the inquiry sequence from Aaron Boley, the instructor for the UBC Astronomy 101 lecture component. We warmly thank all the UBC TAs and PASEA instructors who facilitated the inquiry labs with openness and enthusiasm to learn new ways of teaching, and who provided valuable feedback. We are grateful to E. F. Ladd at Bucknell University for sharing his group’s parallax lab and S3CI questions, which provided inspiration for our Lab A and distance concept inventory questions (as cited in the text). Many thanks to Thai Duy Cuong Nguyen and Alison Rose for beautiful photography and videography at PASEA. And special gratitude to all of our UBC and PASEA students for their curiosity and engagement, and the opportunity to learn from them.
Initial development of the inquiry activity was supported by the Canadian Institute for Theoretical Astrophysics, the Dunlap Institute for Astronomy and Astrophysics, the University of Toronto Department of Astronomy and Astrophysics, and the Institute for Scientist and Engineer Educators at the University of California, Santa Cruz. The UBC adaptation, assessment, and this write-up were supported by the Carl Wieman Science Education Initiative at the University of British Columbia (UBC), including extension funding from John and Deb Harris, the UBC Faculty of Science, and the UBC Department of Physics & Astronomy, as well as a Skylight Development Grant from the UBC Faculty of Science.
We respectfully acknowledge that much of this work (UBC teaching and writing this article) took place on the traditional, ancestral, and unceded territory of the Musqueam, Squamish and Tsleil-Waututh First Nations. J.Z. respectfully acknowledges the Wangal people—the traditional owners of the land upon which lived as she worked on this paper.
References
- Kozminski J, Lewandowski H, Beverly N, Lindaas S, Deardorff D, Reagan A, Dietz R, Tagg R, Eblen-Zayas M, Williams J, Hobbs R, Zwickl B. 2014. AAPT recommendations for the undergraduate physics laboratory curriculum. American Association of Physics Teachers, College Park, MD. https://www.aapt.org/resources/upload/labguidlinesdocument_ebendorsed_nov10.pdf.
- Wieman C. 2015. Comparative cognitive task analyses of experimental science and instructional laboratory courses. Phys Teach 53:349–351. DOI:10.1119/1.4928349.
- National Research Council. 2000. Inquiry and the National Science Education Standards: A guide for teaching and learning. The National Academies Press, Washington, DC. DOI:10.17226/9596.
- Rönnebeck S, Bernholt S, Ropohl M. 2016. Searching for a common ground – A literature review of empirical research on scientific inquiry activities. Stud Sci Educ 52:161–197. DOI:10.1080/03057267.2016.1206351.
- Rafelski M, Foley M, Graves GJ, Kretke KA, Mills E, Nassir M, Patel S. 2010. Teaching astronomy with an inquiry activity on stellar populations, p 108–119. In Hunter L, Metevier AJ (ed), Learning from inquiry in practice, ASP Conference Series. Astronomical Society of the Pacific, San Francisco, CA.
- McConnell N, Medling A, Strubbe L, Raschke L, Hunter L. 2010. A college-level inquiry-based laboratory activity on transiting planets, p 97–107. In Hunter L, Metevier AJ (ed), Learning from inquiry in practice, ASP Conference Series. Astronomical Society of the Pacific, San Francisco, CA.
- Raschke L, Severson S, Seagroves S, Porter J. 2010. The COSMOS Optics Inquiry: An inquiry activity for high school students on geometric optics, p 138–147. In Hunter L, Metevier AJ (ed), Learning from inquiry in practice, ASP Conference Series. Astronomical Society of the Pacific, San Francisco, CA.
- Sonnett S, Mills B, Hamilton JC, Kaluna H. 2010. The 2009 Akamai Observatory short course inquiry activity: “Design and build a telescope,” p 131–137. In Hunter L, Metevier AJ (ed), Learning from inquiry in practice, ASP Conference Series. Astronomical Society of the Pacific, San Francisco, CA.
- Wiggins G, McTighe J. 1998. Understanding by design. Association for Supervision and Curriculum Development, Alexandria, VA.
- Ladd EF, Gingrich EC, Nottis KEK, Udomprasert P, Goodman AA. 2015. Combining real world experiences with WorldWide Telescope visualization to build a better parallax lab, p 191–194. In Greg G, Buxner S, Shore L, Barnes J (ed), Celebrating Science: Putting Education Best Practices To Work, ASP Conference Series. Astronomical Society of the Pacific, San Francisco, CA.
- Strubbe L, Okere BI, Zhang J, Chibueze JO, Ikape M, Okouma PM, Ibik A, White H, Abotsi-Masters S, Man A, Webb S, Asabere BD, Bop CT, Esaenwi S, Mowla L, Nguyen TDC, Odo F, Odoh D, Ofodum CN, Onyeuwaoma N, Cheek W, Deng M, Eze R, Kerzendorf W, Klutse NAB, Lepo K, Pardi AL, Rose A, Urama J. 2021. The Pan-African School for Emerging Astronomers. Nat Astron 5:217–220. DOI:10.1038/s41550-021-01301-7.
- Chinn CA, Malhotra BA. 2002. Epistemologically authentic inquiry in schools: A theoretical framework for evaluating inquiry tasks. Sci Educ 86:175–218. DOI:10.1002/sce.10001.
- Exploratorium Institute for Inquiry. Pathways to learning: The Institute for Inquiry’s approach to teaching and learning science through inquiry. Retrieved from https://www.exploratorium.edu/sites/default/files/pdfs/ifi/Pathways-to-Learning.pdf.
- Hunter L, Metevier AJ (ed). 2010. Learning from inquiry in practice. ASP Conference Series, vol 436. Astronomical Society of the Pacific, San Francisco, CA.
- Kanim S, Cid XC. 2020. The demographics of physics education research. Phys Rev Phys Educ Res 16:20106. DOI:10.1103/PhysRevPhysEducRes.16.020106.
- Brookes DT, Ektina E, Planinsic G. 2020. Implementing an epistemologically authentic approach to student-centered inquiry learning. Phys Rev Phys Educ Res 16:20148. DOI:10.1103/PhysRevPhysEducRes.16.020148.
- Holmes NG, Keep B, Wieman CE. 2020. Developing scientific decision making by structuring and supporting student agency. Phys Rev Phys Educ Res 16:10109. DOI:10.1103/physrevphyseducres.16.010109.
- Smith EM, Stein MM, Walsh C, Holmes NG. 2020. Direct measurement of the impact of teaching experimentation in physics labs. Phys Rev X 10:11029. DOI:10.1103/PhysRevX.10.011029.
- Holmes NG, Wieman CE, Bonn DA. 2015. Teaching critical thinking. Proc Natl Acad Sci 112:201505329. DOI:10.1073/pnas.1505329112.
- Slater SJ, Slater TF, Shaner A. 2008. Impact of backwards faded scaffolding in an astronomy course for pre-service elementary teachers based on inquiry. J Geosci Educ 56:408–416. DOI:10.5408/jge_nov2008_slater_408.
- Buck LB, Bretz SL, Towns MH. 2008. Characterizing the level of inquiry in the undergraduate laboratory. J Coll Sci Teach 38:52–58.
- McDermott LC, the PER Group at the University of Washington. 1996. Physics by inquiry. Wiley, New York, NY.
- French D, Burrows A. 2017. Inquiring astronomy: Incorporating student-centered pedagogical techniques in an introductory college science course. J Coll Sci Teach 46:24–32. DOI:10.2505/4/jcst17_046_04_24.
- Lederman NG, Abd-El-Khalick F, Bell RL, Schwartz RS. 2002. Views of Nature of Science Questionnaire: Toward valid and meaningful assessment of learners’ conceptions of nature of science. J Res Sci Teach 39:497–521. DOI:10.1002/tea.10034.
- Adams WK, Perkins KK, Podolefsky NS, Dubson M, Finkelstein ND, Wieman CE. 2006. New instrument for measuring student beliefs about physics and learning physics: The Colorado Learning Attitudes about Science Survey. Phys Rev ST Phys Educ Res 2:1–14. DOI:10.1103/PhysRevSTPER.2.010101.
- Brewe E, Kramer L, O’Brien G. 2009. Modeling instruction: Positive attitudinal shifts in introductory physics measured with CLASS. Phys Rev ST Phys Educ Res 5:013102. DOI:10.1103/PhysRevSTPER.5.013102.
- Madsen A, McKagan SB, Sayre EC. 2015. How physics instruction impacts students’ beliefs about learning physics: A meta-analysis of 24 studies. Phys Rev ST Phys Educ Res 11:010115. DOI:10.1103/PhysRevSTPER.11.010115.
- Jeffery E, Nomme K, Deane T, Pollock C, Birol G. 2016. Investigating the role of an inquiry-based biology lab course on student attitudes and views toward science. CBE Life Sci Educ 15:ar61. DOI:10.1187/cbe.14-11-0203.
- Lewis SE, Lewis JE. 2005. Departing from lectures: An evaluation of a peer-led guided inquiry alternative. J Chem Educ 82:135–139. DOI:10.1021/ed082p135.
- Laursen SL, Hassi ML, Kogan M, Weston TJ. 2014. Benefits for women and men of inquiry-based learning in college mathematics: A multi-institution study. J Res Math Educ 45:406–418. DOI:10.5951/jresematheduc.45.4.0406.
- Kogan M, Laursen SL. 2014. Assessing long-term effects of inquiry-based learning: A case study from college mathematics. Innov High Educ 39:183–199. DOI:10.1007/s10755-013-9269-9.
- Lombardi D, Shipley TF, Bailey JM, Bretones PS, Prather EE, Ballen CJ, Knight JK, Smith MK, Stowe RL, Cooper MM, Prince M, Atit K, Uttal DH, LaDue ND, McNeal PM, Ryker K, St. John K, van der Hoeven Kraft KJ, Docktor JL. 2021. The curious construct of active learning. Psychol Sci Public Interes 22:8–43. DOI:10.1177/1529100620973974.
- Lock RM, Hazari Z, Potvin G. 2019. Impact of out-of-class science and engineering activities on physics identity and career intentions. Phys Rev Phys Educ Res 15:20137. DOI:10.1103/PhysRevPhysEducRes.15.020137.
- Likert R. 1932. A technique for the measurement of attitudes. Arch Psychol 22:5–55.
- Seagroves S, Hunter L, Palomino R, McConnell N, Metevier A, Barnes A, Quan T. 2018. ISEE’s equity & inclusion theme. Institute for Scientist & Engineer Educators. Retrieved from https://isee.ucsc.edu/about/publications/files/isee_equityinclusion_theme_2018.pdf.
- Schwartz DL, Chase CC, Oppezzo MA, Chin DB. 2011. Practicing versus inventing with contrasting cases: The effects of telling first on learning and transfer. J Educ Psychol 103:759–775. DOI:10.1037/a0025140.
- Stefanou CR, Perencevich KC, DiCintio M, Turner JC. 2004. Supporting autonomy in the classroom: Ways teachers encourage student decision making and ownership. Educ Psychol 39:97–110. DOI:10.1207/s15326985ep3902_2.
- Exploratorium Institute for Inquiry. 2005. Facilitating the focused investigation. Retrieved from http://cfao.ucolick.org/EO/resources/inquiry/FacilitatingHO.pdf.
- Hunter L, Metevier AJ, Seagroves S, Kluger-Bell B, Porter J, Raschke L, Jonsson P, Shaw J, Quan TK, Montgomery R. 2010. Cultivating scientist- and engineer-educators 2010: The evolving professional development program, p 3–49. In Hunter L, Metevier AJ (ed), Learning from inquiry in practice, ASP Conference Series. Astronomical Society of the Pacific, San Francisco, CA.
- Strubbe LE, Stang J, Holland T, Sherman SB, Code WJ. 2019. Faculty adoption of active learning strategies via paired teaching: Conclusions from two science departments. J Coll Sci Teach 49:31–39.
- Bohrer K, Stegenga BD, Ferrier A. 2007. Training and mentoring TAs in inquiry-based methods, p 335–346. In O’Donnell MA (ed), Tested Studies for Laboratory Teaching. Proceedings of the 28th Workshop/Conference of the Association for Biology Laboratory Education (ABLE).
- Hufnagel B. 2002. Development of the Astronomy Diagnostic Test. Astron Educ Rev 1:47–51. DOI:10.3847/aer2001004.
- Gingrich EC, Ladd EF, Nottis KEK, Udomprasert P. 2015. The Size, Scale, and Structure Concept Inventory (S3CI) for astronomy, p 269–273. In Schultz G, Buxner S, Shore L, Barnes J (ed), Celebrating science: Putting education best practices to work. Astronomical Society of the Pacific, Burlingame, CA.
- Nissen JM, Talbot RM, Nasim Thompson A, Van Dusen B. 2018. Comparison of normalized gain and Cohen’s d for analyzing gains on concept inventories. Phys Rev Phys Educ Res 14:10115. DOI:10.1103/PhysRevPhysEducRes.14.010115.
- Kraft MA. 2019. Interpreting effect sizes of education interventions. (Ed WorkingPaper No. 19-10). Retrieved from Annenberg Institute at Brown University: http://www.edworkingpapers.com/ai19-10.
- Schmitt N. 1996. Uses and abuses of coefficient alpha. Psychol Assess 8:350–353. DOI:10.1037/1040-3590.8.4.350.
- Makwela TN. 2022. PhD thesis. Probing student engagement with size and distance in introductory astronomy. University of Cape Town, Cape Town, South Africa.
- Coble K, Camarillo CT, Trouille LE, Bailey JM, Cochran GL, Nickerson MD, Cominsky LR. 2013. Investigating student ideas about cosmology I: Distances and structure. Astron Educ Rev 12. DOI:10.3847/AER2012038.
- Rajpaul VM, Lindstrøm C, Engel MC, Brendehaug M, Allie S. 2018. Cross-sectional study of students’ knowledge of sizes and distances of astronomical objects. Phys Rev Phys Educ Res 14:20108. DOI:10.1103/PhysRevPhysEducRes.14.020108.
- Miller BW, Brewer WF. 2010. Misconceptions of astronomical distances. Int J Sci Educ 32:1549–1560. DOI:10.1080/09500690903144099.
- Zwickl BM, Hirokawa T, Finkelstein N, Lewandowski HJ. 2014. Epistemology and expectations survey about experimental physics: Development and initial results. Phys Rev ST Phys Educ Res 10:010120. DOI:10.1103/PhysRevSTPER.10.010120.
- Lock RM, Hazari Z, Potvin G. 2013. Physics career intentions: The effect of physics identity, math identity, and gender. AIP Conference Proceedings 1513:262–265. DOI:10.1063/1.4789702.
- Godwin A, Potvin G, Hazari Z. 2013. The development of critical engineering agency, identity, and the impact on engineering career choices. Paper presented at the 2013 ASEE Annual Conference & Exposition. American Society for Engineering Education, Atlanta, GA. DOI:10.18260/1-2--22569.
- Quan GM, Elby A. 2016. Connecting self-efficacy and views about the nature of science in undergraduate research experiences. Phys Rev Phys Educ Res 020140:1–14. DOI:10.1103/PhysRevPhysEducRes.12.020140.
- Ryan RM, Deci EL. 2000. Self-determination theory and the facilitation of intrinsic motivation, social development, and well-being. Am Psychol 55:68–78. DOI:10.1037/0003-066X.55.1.68.
- Bandura A. 2001. Social cognitive theory: An agentic perspective. Annu Rev Psychol 52:1–26. DOI:10.1146/annurev.psych.52.1.1.
Article Files
Login to access supporting documents
Strubbe-Good-Zhang-White-Lepo-Code-Abotsi-Masters-Distances in the Universe An Inquiry Lab Sequence Taught in West Africa and North America.pdf(PDF | 2 MB)
S1. Distance Inquiry - Lab A - Lesson Plan.docx(DOCX | 92 KB)
S2. Distance Inquiry - Lab A - TA Meeting Plan.docx(DOCX | 24 KB)
S3. Distance Inquiry - Lab A - Pre-lab.docx(DOCX | 68 KB)
S4. Distance Inquiry Lab A - In-lab worksheet.docx(DOCX | 9 KB)
S5. Distance Inquiry - Lab A - In-lab hint sheets.pptx(PPTX | 40 KB)
S6. Distance Inquiry - Lab A - In-lab Trig Tip Sheet.docx(DOCX | 27 KB)
S7. Distance Inquiry - Lab A - Post-lab - Worksheet version - Hand out during lab.docx(DOCX | 9 KB)
S8. Distance Inquiry - Lab A - Post-lab - Version to post after class on LMS.docx(DOCX | 14 KB)
S9. Distance Inquiry - Lab A - Pre-lab Answers.docx(DOCX | 68 KB)
S10. Distance Inquiry - Lab A - In-lab worksheet - Rubric.docx(DOCX | 9 KB)
S11. Distance Inquiry - Lab A - Post-lab - Answer key and rubric.docx(DOCX | 15 KB)
S12. Distance Inquiry - Lab A - Post-lab - Answer key to share with students.docx(DOCX | 15 KB)
S13. Distance Inquiry - Lab B - Lesson Plan.docx(DOCX | 687 KB)
S14. Distance Inquiry - Lab B - TA Meeting Plan.docx(DOCX | 12 KB)
S15. Distance Inquiry - Lab B - Prelab with answers.docx(DOCX | 18 KB)
S16. Distance Inquiry - Lab B - Worksheet 1 - Sedna.docx(DOCX | 11 KB)
S17. Distance Inquiry - Lab B - Worksheet 2 - Shadow puppets.docx(DOCX | 9 KB)
S18. Distance Inquiry - Lab B - Worksheet 3 - Hemispheres.docx(DOCX | 8 KB)
S19. Distance Inquiry - Lab B - In-lab Light rays hint sheet.pdf(PDF | 230 KB)
S20. Distance Inquiry - Lab B - In-lab List of constants.docx(DOCX | 8 KB)
S21. Distance Inquiry - Lab B - Post-lab.docx(DOCX | 10 KB)
S22. Distance Inquiry - Lab B - Worksheet 1 - Sedna - Answer Key.docx(DOCX | 12 KB)
S23. Distance Inquiry - Lab B - Worksheet 2 - Shadow puppets - Answer Key.docx(DOCX | 279 KB)
S24. Distance Inquiry - Lab B - Worksheet 3 - Hemispheres - Answer Key.docx(DOCX | 9 KB)
S25. Distance Inquiry - Lab B - Post-lab - Answer key and rubric.docx(DOCX | 11 KB)
S26. Distance Inquiry - Lab C - Lesson Plan.docx(DOCX | 18 KB)
S27. Distance Inquiry - Lab C - TA Meeting Plan.docx(DOCX | 11 KB)
S28. Distance Inquiry - Lab C - Pre-lab.docx(DOCX | 10 KB)
S29. Distance Inquiry - Lab C - In-lab worksheet.docx(DOCX | 8 KB)
S30. Distance Inquiry - Lab C - Post-lab.docx(DOCX | 8 KB)
S31. Distance Inquiry - Lab C - Pre-lab - Answer key.docx(DOCX | 10 KB)
S32. Distance Inquiry - Lab C - In-lab worksheet - Rubric.docx(DOCX | 8 KB)
S33. Distance Inquiry - Lab C - Post-lab - Rubric.docx(DOCX | 9 KB)
S34. Distance Inquiry - Lab C - Slide deck.pptx(PPTX | 613 KB)
S35. Distance Inquiry - Lab C - Starter images.pptx(PPTX | 12 MB)
S36. Distance Inquiry - Lab D - Lesson Plan.docx(DOCX | 19 KB)
S37. Distance Inquiry - Lab D - TA Meeting Plan.docx(DOCX | 10 KB)
S38. Distance Inquiry - Lab D - In-Lab _ Post-Lab Worksheet.docx(DOCX | 10 KB)
S39. Distance Inquiry - Lab D - Presentations Assignment in preparation for Lab E.docx(DOCX | 9 KB)
S40. Distance Inquiry - Lab D - In-Lab _ Post-Lab Worksheet - Answer key and rubric.docx(DOCX | 21 KB)
S41. Distance Inquiry - Lab D - Slide deck.pptx(PPTX | 491 KB)
S42. Distance Inquiry - Lab E - Lesson Plan.docx(DOCX | 17 KB)
S43. Distance Inquiry - Lab E - TA Meeting Plan.docx(DOCX | 9 KB)
S44. Distance Inquiry - Lab E - In-lab worksheet - Audience notes.docx(DOCX | 9 KB)
S45. Distance Inquiry - Lab E - Lab Report Assignment.docx(DOCX | 10 KB)
S46. Distance Inquiry - Lab E - Presentation Scoring sheet.docx(DOCX | 9 KB)
S47. Distance Inquiry - Lab E - Presentation Scoring sheet - Notes for Facilitators.docx(DOCX | 11 KB)
S48. Distance Inquiry - Lab E - In-lab worksheet - Rubric.docx(DOCX | 8 KB)
S49. Distance Inquiry - Lab E - Goals for lab report.docx(DOCX | 8 KB)
S50. Distance Inquiry - Lab E - Lab Report - Rubric.docx(DOCX | 10 KB)
S51. Distance Inquiry - Lab E - Slide deck.pptx(PPTX | 469 KB)
S52. Distance Inquiry - Pre-semester TA Meeting Plan.docx(DOCX | 17 KB)
S53. Distance Inquiry - Facilitator reflection prompts.docx(DOCX | 9 KB)
S54. Distance Inquiry - Notes for grading inquiry labs.docx(DOCX | 9 KB)
S55. Distance Inquiry - Questions for students after labs A B E.docx(DOCX | 10 KB)
S56. Distance Inquiry - Inquiry Labs overview - for students.docx(DOCX | 8 KB)
S57. Distance Inquiry - Distance concept inventory questions and analysis.docx(DOCX | 12 KB)
S58. Distance Inquiry - Lab D - Parallax Type 1 - Facilitation guide short version additional notes.docx(DOCX | 121 KB)
S59. Distance Inquiry - Lab D - Parallax Type 1 - Facilitation guide full version.pdf(PDF | 2 MB)
S60. Distance Inquiry - Lab D - Parallax Type 1 - Description of the simulated data.docx(DOCX | 9 KB)
S61. Distance Inquiry - Lab D - Parallax Type 2 - Facilitation guide.docx(DOCX | 42 KB)
S62. Distance Inquiry - Lab D - Parallax Type 2 - Description of the simulated data.docx(DOCX | 40 KB)
S63. Distance Inquiry - Lab D - Parallax Type 2 - Data - 51Peg_Stellarium.png(PNG | 37 KB)
S64. Distance Inquiry - Lab D - Parallax Type 2 - Data - 51Peg_Stellarium_shifted.png(PNG | 21 KB)
S65. Distance Inquiry - Lab D - Parallax Type 2 - Data - Fomalhaut_Stellarium.png(PNG | 42 KB)
S66. Distance Inquiry - Lab D - Parallax Type 2 - Data - Fomalhaut_Stellarium_shifted.png(PNG | 24 KB)
S67. Distance Inquiry - Lab D - Parallax Type 2 - Data - Gliese445_Stellarium.png(PNG | 42 KB)
S68. Distance Inquiry - Lab D - Parallax Type 2 - Data - Gliese445_Stellarium_shifted.png(PNG | 26 KB)
S69. Distance Inquiry - Lab D - Parallax Type 2 - Data - ProximaCen_Stellarium.png(PNG | 79 KB)
S70. Distance Inquiry - Lab D - Parallax Type 2 - Data - ProximaCen_Stellarium_Shifted.png(PNG | 45 KB)
S71. Distance Inquiry - Lab D - ISL Kepler stars - Facilitation guide.docx(DOCX | 14 KB)
S72. Distance Inquiry - Lab D - ISL Kepler stars - Data - Set of template stellar spectra - For students.docx(DOCX | 117 KB)
S73. Distance Inquiry - Lab D - ISL Kepler stars - Data - Set of template stellar spectra - Answer key.docx(DOCX | 9 KB)
S74. Distance Inquiry - Lab D - ISL Kepler stars - Data - Kepler 20 Spectrum.docx(DOCX | 24 KB)
S75. Distance Inquiry - Lab D - ISL Kepler stars - Data - Kepler 20 Light curve.docx(DOCX | 62 KB)
- License terms
Comments
Comments
There are no comments on this resource.