Snail Races: An Inquiry-Based Approach to Learn Invasive Species Ecology
Editor: Jennifer Momsen
Published online:
Abstract
Inquiry-based lessons allow learning that is hands-on and student-driven, fostering engagement and retention of knowledge in any discipline. Here, we use this learning framework to engage students in exploring the effects of biotic and abiotic factors on animal dispersal as a means of connecting students to multiple ecological concepts. Instructors are provided with a guided lecture on key ecological concepts including the impacts of invasive species, dispersal mechanisms, and species interactions with the environment. Furthermore, the interactive lecture introduces students to the snail study system and explains the experimental process, which involves low-cost, readily available materials (e.g., kiddie pools). Students design experiments that explore how variables including density, substrate, and temperature can affect animal dispersal. They conduct the experiments, engage in a process of peer-review, analyze results, and share their study. We performed this lesson in an undergraduate Ecology course and report here on student responses to the experience. The lesson is designed to be performed with the faucet snail (Bithynia tentaculata), an invasive freshwater species throughout much of the Great Lakes region, but can be easily modified to accommodate other species. The lesson is also highly amenable to modifications for variable class sizes, student grade levels, and lesson duration.
Primary Image: An adult faucet snail (Bithynia tentaculata) resting on submerged aquatic vegetation. The faucet snail (named after its ability to clog faucets and drainages) is native to Europe but invaded the Great Lakes of North America in the late 1800s. The snail is host to several species of parasitic trematodes that can cause high rates of mortality in waterfowl, following the consumption of infected snails.
Citation
Grindle E, Bergan-Roller HE, Johnson A, Lunaburg G, Koop JAH. 2023. Snail Races: An Inquiry-Based Approach to Learn Invasive Species Ecology. CourseSource 10. https://doi.org/10.24918/cs.2023.47Society Learning Goals
Ecology
- Species-Habitat Interactions
- How do species interact with their habitat?
- Interactions within Ecosystems
- How are living systems interconnected and interacting?
- Impacts of Humans on Ecosystems
- What impacts do humans have on ecosystems?
Lesson Learning Goals
Students will:- apply and use the scientific method.
- assess and confirm the importance of experimental design in testing a hypothesis.
- evaluate how animal dispersal is affected by biotic and abiotic variables.
- create connection and engagement with ecological concepts and relate how humans affect ecosystems.
Lesson Learning Objectives
Students will be able to:- collaboratively design and conduct dispersal experiments.
- problem-solve through peer consult and pilot trials of experiment.
- analyze data to support or refute dispersal-related hypotheses.
- summarize and communicate scientific findings to a general audience.
Article Context
Course
Article Type
Course Level
Bloom's Cognitive Level
Vision and Change Core Competencies
Vision and Change Core Concepts
Class Type
Class Size
Audience
Lesson Length
Pedagogical Approaches
Principles of How People Learn
Assessment Type
Introduction
Authentic science inquiry, including Course-based Undergraduate Research Experiences (CUREs), has become a mainstay of scientific teaching pedagogy (1–3). This approach to teaching involves student-led inquiry that fosters the exploration of a subject through hands-on learning and prioritizes scientific reasoning as a learning outcome (4). Regardless of background, students are provided the same opportunities to experience applied lab work and scientific inquiry, improving inclusivity in the science classroom (5). The inquiry-based lesson framework increases knowledge retention and promotes self-efficacy and confidence in students (6, 7). Furthermore, these types of lessons do not guarantee successful experiments. Students often see their work fail, but are given the opportunity to reflect, redesign, and reimplement a modified methodology (8, 9), engaging with multiple dimensions of scientific inquiry. While formal CUREs are often full semester experiences, we have used this framework to create an inquiry-based lesson of 6–8 hours (2–3 typical undergraduate level lab periods) to explore the effects of biotic and abiotic factors on animal dispersal. The lesson introduces students to multiple ecological concepts including species’ interactions with their environment and the impact of invasive species on native ecosystems. The shorter timeline of the lesson, relative to a formal CURE, allows for implementation in a variety of classroom types.
Invasive species are those that move outside of their native range, establish reproductive populations, and spread beyond the initial point of introduction. Invasive species cause harm to native ecosystems through multiple means including predation, competition with native species, the spread of disease, and even extinction (10–12). These interactions can lead to decreased biodiversity and ecosystem instability. The negative consequences of invasive species and the high frequency with which invasions occur have made the control and management of invasive species a necessary priority for conservation-related organizations (13, 14). Aquatic invasive species present a particular challenge to manage in part because they are relatively inconspicuous compared to terrestrial species and often have dispersal mechanisms that allow them to spread rapidly (15–17). For example, the zebra mussel (Dreissena polymorpha) was accidentally introduced through ship ballast water from Europe into Lake St. Clair, near Detroit, MI, in 1986 (18). Zebra mussels are remarkably prolific and cause damage to natural and human-made structures through biofouling. They can also destabilize food web structures by altering phytoplankton composition (19–21). The ability of zebra mussels to attach to ships and recreational fishing boats facilitated their dispersal throughout most central and eastern United States waterways in less than a decade (USGS Nonindigenous Aquatic Species Database).
Dispersal of aquatic species can occur passively, for example, by being transported by boats or birds, or actively, for example, crawling, swimming, or riding currents. Active dispersal mechanisms are subject to variation depending on the conditions directly experienced by dispersing organisms (22, 23). Biotic and abiotic conditions such as temperature, vegetative cover, infection status, flow velocity, and population density, can alter the speed at which active dispersal occurs (24, 25). Therefore, understanding species interactions with their environment and how those interactions change dispersal patterns at a local scale can translate into more accurate predictions of colonization ability and broad-scale species distributions (26). Here, we present a lesson to investigate the role of biotic and abiotic conditions on the micro-scale active dispersal behaviors of an aquatic invasive snail.
System Background
Faucet snails (Bithynia tentaculata) are small freshwater snails native to Europe and likely introduced through ship ballast water to North America (27). While the faucet snail is quite small, only reaching a maximum of approximately 12–15mm shell length, they are highly prolific. In fact, their common name comes from their potential to clog faucets and drainages through the rapid accumulation of individuals (28). According to natural history observations, the faucet snail was first observed in the Great Lakes region in the late 1800s and has since made its way westward across the Great Lakes into the Upper Mississippi River where it is currently moving southward (27).
The faucet snail is host to several parasitic trematode species that complete their life cycle in waterfowl that have consumed an infected snail (29, 30). The trematodes develop into adult worms in the intestines of birds and can cause lethal intestinal bleeding. Tens of thousands of waterfowl, especially including Lesser Scaup (Aythya affinis) and American Coot (Fulica americana), have died because of trematode infection in the upper Mississippi River region (31). For the purposes of this lesson, it is important to note that these trematode species do not infect humans and thus the snails do not require special protocols for handling. Studies on the infection patterns (30, 32–35) and population dynamics (36, 37) of this system are increasing, but studies investigating how biotic and abiotic factors affect small-scale dispersal behavior of the snail are still needed. A better understanding of how snails disperse at a local scale will help more accurately predict the invasive potential of the snail and trematode parasites. Here, we propose a lesson to address this gap in knowledge. This work can be done in a variety of classroom settings with low-cost, accessible materials.
Intended Audience
This lesson is highly adaptable for students ranging from middle school to upper-level undergraduates. We originally implemented this lesson in a course for undergraduate biology students with an interest in ecology. Prerequisite courses included introductory general biology courses. The class size was 24 students who were organized into 6 groups.
Required Learning Time
This lesson was designed to take 6–8 hours, which aligns with 2–3 typical undergraduate course lab periods. Our course met once per week, so, in this case, the lesson was carried out over the course of two weeks (i.e., one 3-hour lab period each week). Student work outside of class time averaged one and a half hours per week. If additional components, such as extensive literature searches, are included, instructors may need to account for extra time (e.g., another lab period). We asked students to find 2–3 scientific articles that helped justify their hypothesis. They were able to do this outside of class time and between the two meeting periods. Similarly, if student presentations are included, instructors should plan on at least one additional, 3-hour lab period. Presentation length can range based on group size, but we recommend 12–15 minutes per group.
Prerequisite Student Knowledge
Students are expected to have an introductory understanding of biology and ecology. In addition, it is recommended that students have a familiarity with the scientific method (38) and some prior experience finding and reading primary literature (39). Through the provided introductory lecture (Supporting File S4), the lesson introduces students to the more specific major concepts of the lab prior to starting, including passive and active dispersal mechanisms, biotic and abiotic variables, and defining invasive species and their effects on native ecosystems.
Prerequisite Teacher Knowledge
Instructors should be familiar with how invasive species harm native ecosystems (e.g., predation, competition, disease). We recommend using local, recent examples whenever possible to better engage students. We expect instructors to give a general overview of active and passive dispersal mechanisms and provide students with examples of biotic and abiotic factors that may affect dispersal. Instructors will need a basic knowledge of the ecology and natural history of the aquatic species they choose to use for the lab. All information is included in the Instructor Packet (Supporting File S1) and the Instructor Prelab Presentation (Supporting File S4). Instructors wanting to require students to include data analysis or graphical representations of results can make use of the sample data set and analysis resource (Supporting File S3).
Scientific Teaching Themes
Active Learning
Student-directed learning promotes ownership of the learning experience and fosters student engagement. LaDue and colleagues (40) presented active learning as involving engagement in four categories: behavioral, agentic, emotional, and cognitive. To briefly define these terms, behavioral engagement refers to visible effort made by students (e.g., participation in discussions, completion of worksheets, etc.). Agentic engagement refers to student-driven questioning and leading of their own learning process. Emotional engagement refers to students’ sense of belonging, interest, or value in a class. Cognitive engagement refers to self-regulation and motivation in terms of engaging with the material through thinking patterns and strategies. This lesson is intended to be a fully hands-on experience from start to finish requiring or encouraging all four categories of active learning. For example, students receive worksheets to guide the development of questions and hypotheses (i.e., behavioral engagement). Students then design their own experiment, consult with peers, and conduct trials (i.e., agentic and cognitive engagement). Following data analysis and presentation (either in written or presentation form), guided reflection exercises again touch on all four types of engagement (i.e., behavioral, agentic, emotional, and cognitive engagement).
Assessment
The basics of assessment for this lesson include student participation in all lab activities (may be measured by completion of the Student Packet [Supporting File S2]). The students may also be assessed on their participation in peer consultation and data collection. Additional assessments for a written summary or in-class presentation are provided and encouraged but require student work outside of the lab or additional lab periods to complete. Both assessments instruct students to find supporting scientific literature to justify their study and to place their results within a larger biological context. We ask students to critically reflect on their own hypotheses and experimental design. We have provided a rubric for the written summary and presentation that draw from key learning objectives. These rubrics will also contain sections to assess student participation both in their own groups and in the context of providing peer consultation to other groups.
Inclusive Teaching
Inquiry-based lessons as a learning framework are found to promote diversity, equity, and inclusion (5, 41). They allow all students in a course to have a hands-on research experience which may have previously only been available to more privileged students who get accepted into research labs. From the instructor perspective, inquiry-based lessons promote the mindset that all students in a class can succeed given the right tools (42). This lesson is largely student-driven making it widely adjustable to varying levels of knowledge and experience. The amount of instructor oversight is also highly adaptable. We encourage instructors to improve inclusion by asking students to share their answers to the final question on the guided worksheet. This question asks students to reflect on their favorite part of the exercise, highlighting their most meaningful contribution to the project (e.g., exploring the question, designing or redesigning the experiment, analyzing the data, communicating the results to a broader audience, improving group work dynamics, or acting as a leader to assign tasks and improve work efficiency). Shared experiences and contributions help reinforce each student’s sense of belonging.
Lesson Plan
Pre-Class Preparation
Instructors can either collect snails prior to the lesson or include students in the excursion depending on available resources and accessibility. For our implementation of this lesson, we collected snails and kept them in tanks prior to student use. Instructors will need to estimate the number of snails needed. For example, if there are 4 student groups, 2 pools/group (8 total), 5 trials/group, 20 snails per trial, then approximately 800 snails would be needed, assuming students elected to start with new snails for each trial. Faucet snails are found throughout much of the Great Lakes region and often in very high densities. In many locations, 800 snails could be collected within an hour. Instructors may use the map provided by USGS which lists dates and locations where faucet snails have been found. Instructors should also feel free to contact the corresponding author with questions about collection locations or techniques.
Faucet snails are generally not larger than 15mm in length and have a shiny brown shell with a rounded spire and an operculum with concentric rings (43) (Figure 1). Faucet snails are most often found along rocky shorelines with nearby vegetation such as wild celery beds. The snails can be collected by hand from the surface of submerged rocks and by brushing along submerged plant stems. Snails can be transported in plastic pails or containers filled with water from the collection site. It is important to keep snails out of direct sunlight during transport to not overheat them. Snails will survive in plastic pails or aquariums at room temperature. If additional water is needed or snails are being kept for more than a few days, regular water changes with de-chlorinated tap water should be performed at least weekly. An aeration stone also helps to keep the water oxygenated. Snails kept for more than a few days will require food. For short term options (i.e., 5–7 days) we recommend green leaf lettuce leaves or algae wafers (e.g., Tetra® PlecoWafers). In the case of keeping snails for more than 7 days, a food mixture of BD Bacto™ Agar and dried lettuce is needed. Instructions for preparing that food are in Instructor Packet (Supporting File S1).
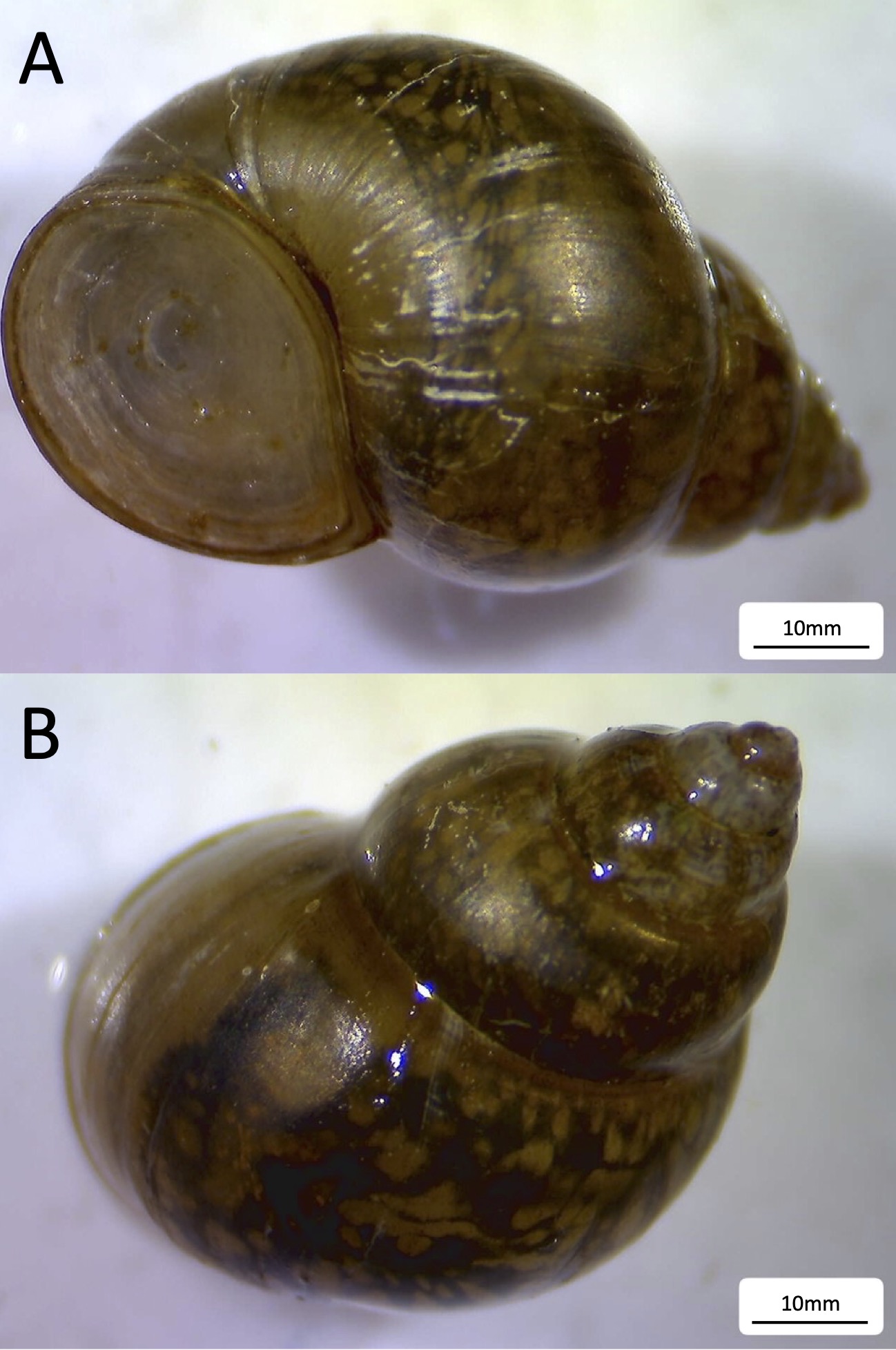
Instructors using this lesson outside the geographic range of faucet snails will need to substitute another snail species. Many species of aquatic snails are also easily kept in the conditions described above, especially for short periods of time. Aquatic snails are ideal for this lesson since they provide an opportunity for students to get hands-on experience with animals without significant prior training. Care should be taken to check for any necessary permits required to collect protected, native snail species. Since snails are invertebrates, Institutional Animal Care and Use Committee (IAUCU) permits are not required. We have provided several useful links for identifying aquatic snail species in North America (Supporting File S1).
Instructors should set up kiddie pools (~1 meter diameter) ahead of time to ensure that there are no leaky or faulty pools. Fill pools with de-chlorinated tap water to a depth of 10–20 cm. Instructors can use regular tap water but we recommend a de-chlorination treatment such as Tetra® AquaSafe Water Conditioner.
Instructors should familiarize themselves with all the materials (both written handouts and equipment) before running the experiment. We recommend at least one pilot trial prior to involving students to familiarize instructors with the full protocol. For adult faucet snails, trial times of 30–60 minutes allowed for snails to move from the pool center to the perimeter. If using a different snail species, more or less time may need to be allotted for trials to optimize data collection (i.e., provide snails sufficient time to move a measurable distance but avoid excessive movement such that tracking becomes difficult).
Prior to class prepare the handouts, including the Student Packet (Supporting File S2). Instructors should feel free to revise the Guided Worksheet for Students to include the questions that are most relevant to their learning goals. For example, if no statistical analyses will be completed, these questions should be removed from the worksheet template. During the experiment, students will need a writing implement, hard surface to write on (e.g., clipboard, hardcover notebook, etc.), a metric measuring tape, and a device with time keeping abilities (e.g., smartphone, watch, stopwatch).
In-Class Activities
Introductory Lecture (30–40 minutes)
Instructors should provide a short lecture that introduces students to the overall structure of the experiment and necessary background on the study species. In our implementation, the presentation placed initial emphasis on the benefits of inquiry-based lessons in improving student engagement and knowledge retention. We introduced the topics of invasive species and dispersal mechanisms, moving from term definitions to the ecological importance of these topics and finally to the specific study system used for this lesson—mirroring the general information presented in the Introduction section above. Finally, we reviewed the scientific method, including a general overview of the steps to complete the experiment and the variables students can manipulate. We encourage instructors to allow time for students to ask questions during and after the lecture.
Brainstorming (20–40 minutes)
Students work in pairs or small groups (no larger than groups of 5) to determine which biotic or abiotic variables affecting small-scale dispersal they want to test (Table 1). Students draw on information shared in the introductory lecture to generate and justify hypotheses. The resources made available by the instructor will limit the types of hypotheses that students generate, so it is necessary to make students aware of what materials are available to them prior to this step. At more advanced levels, students should justify their hypotheses and predictions with sound biological reasoning procured from an exploration of scientific literature. Having well-reasoned hypotheses and predictions will be especially important in helping students reflect upon and interpret their results. In instances where full-class synchrony or materials are limited, it may be useful for the instructor to choose a focal variable to be tested but still ask students to write and justify predictions. For example, the instructor could identify temperature as the variable to be tested. Students would then need to predict whether snails will move faster in warmer or colder temperatures, what those temperatures will be, and justify their predictions using previously published studies or observations. Classes for which statistical analysis of the data are planned should ask students to draw a figure of their expected result to further clarify their reasoning.
Table 1. Timeline for snail lesson. The lesson is presented as pre-class preparation tasks for the instructor, followed by in-class activities for the students.
Activity | Description | Estimated Time | Notes |
---|---|---|---|
Pre-Class Preparation | |||
Find and collect snails |
|
Variable; should plan for several days and possibly multiple outings searching for snails if instructor is not aware of a location that consistently has snails |
Collection note: Do not work alone. For safety always have at least one other person present. Refer to Supporting File S1. |
Print handouts |
|
5–10 minutes | See Supporting Files S2, S5, and S6. |
Prepare supplies |
|
10–15 minutes | Pools can be set up during the lab period or prior depending on available space. |
Practice trial |
|
30 minutes | If a different mollusk species was chosen, plan on extra time to determine optimal time period to allow the mollusks to disperse before measurement. |
In-Class Activities | |||
Introductory lecture and brainstorming |
|
10 minutes (optional student pre-survey), 50–80 minutes (guided lecture is 30–40 minutes, brainstorming is 20–40 minutes) |
If instructor chooses to collect student experience/opinion data, the pre-survey should be completed by students prior to starting the guided lecture introduction. |
Student trial run and peer consult |
|
30–80 minutes (30–60 minutes for trial run, 20 minutes for peer consult) | For more structured lessons, instructor can assign a trial length and remove this as a discussion variable for students. |
Conduct trials |
|
1 hour minimum, up to 4 hours | Ensure students decide on roles (at least one observer and one recorder). |
Data input and analysis |
|
40 minutes | Data entry can be done outside of class time if necessary (with instructor providing directions either in class or as a recording/handout). |
Reflection |
|
20 minutes | Class discussion is highly recommended for reflection. Discussion guided by Guided Worksheet for Student-led Experiment in Supporting File S2. |
Student presentations or reports (optional) |
|
Variable depending on number of student groups and type of final product; allow 10 minutes for student post-survey (optional) | Assessment options/rubrics – see Supporting File S1; If instructor chooses to collect student experience/opinion data, the post-survey should be completed by students following the final activity. |
Student Trial Run(s) (30–60 minutes)
The instructor will give a brief overview of how to run a pilot trial either interacting with the actual materials and snails present or through verbal explanation. While 30–60 minutes may be necessary for a full trial, instructors may choose a shorter run time (e.g., 10 minutes) for the pilot trials. Students should be clear on the general expectations and the roles that they will need to play during the trial before they do any hands-on work themselves. Students should run at least one pilot trial (including all the steps even if time allotted is minimized) to get an understanding of the entire process.
Peer Consult (20 minutes)
After the pilot trial is complete, students will review their work with peers using the Student Packet (Supporting File S2). Peer review involves pairing groups of students to evaluate the work of others and troubleshoot potential problems. The discussion can be used to review justifications for predictions, identify possible shortcomings of the methods, and, in the case of collaborative data collection, ensure a standardized methodology is being used by all groups. Instructors should encourage students to discuss the importance of replicating their trials to improve the reliability of data and avoid erroneous conclusions (i.e., Type I [false positive] and Type II [false negative] errors). If time is a limitation on the number of replicates any one group can perform, then combining data across groups is an alternative solution. At the instructor’s discretion, a full class discussion may also be necessary to facilitate additional problem solving.
Conduct Trials (1 hour minimum, up to 4 hours)
The number of trials students complete will largely depend on the amount of time allotted for the lab and the number of pools available for each group. Student groups should be allowed to work independently to follow their methodology and collect the data they deemed necessary. It is extremely important that instructors not intervene too heavily at this stage, allowing students to make small errors that they can reflect on later.
Data Input and Analysis (40 minutes)
After completion of the full trials, students should input their data into an electronic format (e.g., Microsoft Excel). Students should keep a hard copy of their data and take care to ensure accurate data entry. Generally, pairs are optimal for this stage to keep all students engaged in the activity, but this can be modified depending on class size. The instructors provide guidance in completing statistical analyses. Depending on student skill level, students could calculate and compare the mean distance traveled by the experimental and control groups, as well as the standard deviation, and standard error for each group. The study has been designed to allow for various levels of analysis. Students can compare the means of each group graphically using bar or box plots. If statistical significance is to be tested, the study design dictates that a t test (or Mann-Whitney U test) should be sufficient for comparing means. Instructors may need to provide guidance to complete these tests, though simple internet searches are usually sufficient to guide undergraduate level students through the process. All tests can be done in programs such as Microsoft Excel (with the Data Analysis ToolPak add-on). We encourage instructors to prompt students with questions about the biological significance of their results as well as the statistical significance (e.g., “Even if your group obtained a statistically significant difference between the two treatment means, do you think that difference has real biological meaning?” or “Do you think the difference you observed in your experiment translates into real world implications for snails in the wild? Why?). We found this question to be particularly thought provoking for students.
Reflection (20 minutes)
A recap in the form of a brief class discussion or by completing the “Discussion and Reflection” questions in the Student Packet (Supporting File S2) provides the opportunity for students to reflect on their overall experience.
Additional Assessments: Student Presentations or Reports (5 minutes)
Depending on whether instructors elect to use an additional assessment tool, this time is used to explain the assignment to students. We have provided rubrics for two additional assessments: a written summary or an oral presentation (Assessment Rubrics in Supporting File S1). The lesson in its current form does not include time for student presentations. Including this option would require additional class time.
Teaching Discussion
Modifications and Extensions
This lesson was designed such that adjustments can easily be made to accommodate variable class time, desired skill sets for students, and grade level. The modularity of the lesson design allows for it to take place during multiple short class periods (40–50 minutes) or several longer lab periods (3–4 hours). However, the lesson may also be extended over the course of an entire semester (see “Modifications and Extensions”). Again, depending on desired learning objectives, additional time can be spent to emphasize particular skill sets. For example, instructors may ask students to find and present relevant current scientific research related to their proposed hypothesis. In this case, a full lab period may be dedicated to helping students use search tools for scientific literature and instructing students on the most efficient means of reading scientific papers for comprehension (39). If time allows, instructors may want to provide their students with the opportunity to complete the full lesson, reflect, then revise their hypothesis or methods, and rerun the experiment, as iteration is an important practice of science inquiry and has a positive impact on student learning and experience (1). Alternatively, instructors wanting to build statistical knowledge among their students may want to devote several lab periods to describing the appropriate use of various statistical tests. Finally, instructors wanting to build writing skills among their students could focus several lab periods on scientific writing and communication, peer editing, and revision processes.
The lesson is also adaptable to both introductory and upper-level courses. In an introductory-level setting, the instructor should pare down options of biotic and abiotic variables and provide some oversight in designing methodology. In an upper-level course, students can be given more autonomy to design and lead their own experiments. The background information provided to students should also be tailored to the level of the class. To promote foundational comprehension and knowledge retention, introductory-level classes can use the faucet snail system to connect broad concepts of invasive species and dispersal to a real-world example. In upper-level classes, students should be encouraged to research how local scale mechanisms of dispersal translate to broader geographic patterns. Here, students are guided in learning how to critically analyze new information to fit into or change their current understanding of a topic, which is a highly relevant skill for students as they advance in any field of study.
Lesson Effectiveness
This lesson was tested with a single cohort of twenty-four students in the fall of 2022. Students in a General Ecology course designed for sophomore and junior level undergraduates majoring in Biology or Environmental Studies were asked to take part in surveys that gauge students’ experiences and perceptions (Supporting Files S5, S6). Students took a pre-survey prior to participating in any aspect of the lesson, then took a post-survey after they completed the presentation of their results (the last component of the lesson). Both pre and post-surveys were modified from the Grinnell College CURE survey. Twenty-four students participated in the pre-survey and 20 students participated in the post-survey. Of the 20 students who participated in the post-survey, 70% (14 of 20) self-identified as “female” and 30% (6 of 20) as “male.” In addition, 75% self-identified as “white,” 25% cumulatively self-identified as either “Hawaiian,” “Other,” “Black or African American,” “Filipino,” or “Two or more races.” The first portion of the survey assessed students’ experience with inquiry-based lessons and implementing the scientific method first-hand. The second portion of the survey assessed student perceptions and opinions of self-efficacy, including confidence in studying science and sense of belonging in science. Following participation in the lesson, we asked students to repeat the survey to evaluate changes in responses. The only difference between the two surveys was the request for demographic information in the post participation survey. We analyzed the data from both surveys to gain insight on students’ experiences and perceptions of self-efficacy.
While the goal of this study was not to perform a rigorous assessment of the role of inquiry-based lessons in changing student experience and perceptions of self-efficacy, students did report several shifts from pre- to post-survey answers. Most notably, we observed increases in how much experience students had with performing an experiment entirely of student design (Wilcoxon matched-pairs signed rank test, n = 20, p < 0.001) and in presenting their results in written form (n = 20, p = 0.04) (Figure 2). There were no major shifts in student perceptions following participation in the lesson; however, many of these students were junior and senior undergraduate level. Thus, their perceptions of science may be less likely to shift based on a single additional experience. Still, pre- and post-survey answers of scientific perceptions generally supported the desired learning outcomes (Figure 3). For example, students agreed with statements of self-efficacy (e.g., “Even if I forget the facts, I’ll still be able to use the thinking skills I learn in science.” “I can do well in science courses.” “Explaining science ideas to others has helped me understand the ideas better.”). Overall, we see this lesson as a successful tool to teach students about concepts in ecology and invasion biology, while simultaneously gaining experience with scientific inquiry and improving or reinforcing student self-efficacy.
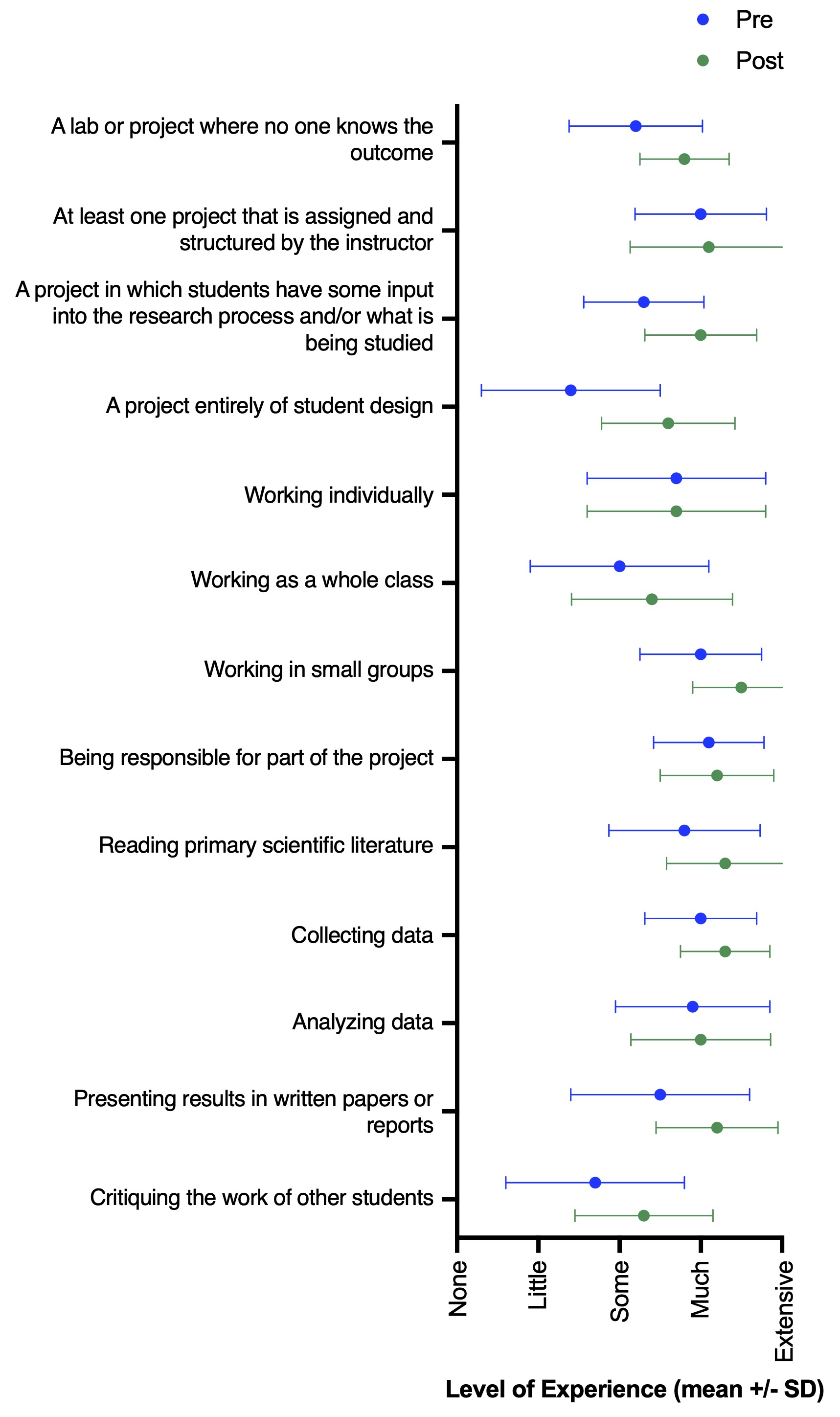
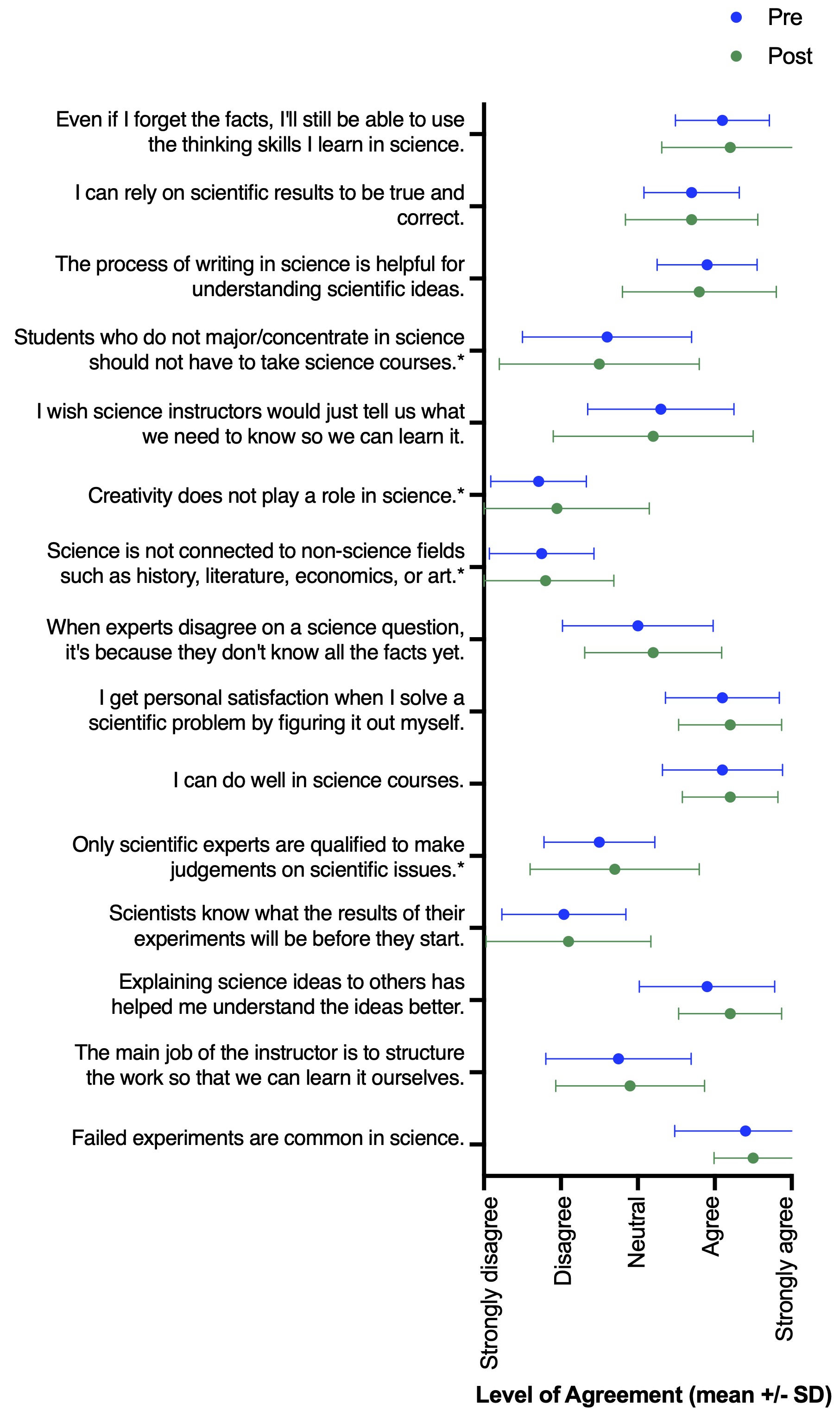
Supporting Materials
-
S1. Snail Races – Instructor Packet
-
S2. Snail Races – Student Packet
-
S3. Snail Races – Sample Data Sheet for Analysis
-
S4. Snail Races – Instructor Prelab Presentation
-
S5. Snail Races – Student Pre-survey
-
S6. Snail Races – Student Post-survey
Acknowledgments
We thank the students of General Ecology, Fall 2022, for their participation in and feedback on this lesson. We thank Craig Schultz, Greenhouse Manager at NIU, for help in setting up space for the students to conduct their experiments. We thank Chloe Gherardi, graduate teaching assistant, for her help in guiding students. We thank members of the Koop and Bergan-Roller labs for their valuable feedback on the manuscript. Funding was provided by Northern Illinois University through a Great Journeys Fellowship to J.A.H. Koop and through a Smerge Scholarship to A. Johnson and G. Lunaburg. This work was approved by the Northern Illinois University Institutional Review Board (IRB HS23-0053, approved August 24, 2022).
References
- Buchanan AJ, Fisher GR. 2022. Current status and implementation of science practices in course-based undergraduate research experiences (CUREs): A systematic literature review. CBE Life Sci Educ 21:ar83. doi:10.1187/cbe.22-04-0069.
- Woodin T, Carter VC, Fletcher L. 2010. Vision and change in biology undergraduate education, a call for action—Initial responses. CBE Life Sci Educ 9:71–73. doi:10.1187/cbe.10-03-0044.
- National Academies of Sciences, Engineering, and Medicine. 2015. Integrating discovery-based research into the undergraduate curriculum: Report of a convocation. The National Academies Press, Washington, DC.
- Chinn CA, Malhotra BA. 2002. Epistemologically authentic inquiry in schools: A theoretical framework for evaluating inquiry tasks. Sci Educ 86:175–218. doi:10.1002/sce.10001.
- Bangera G, Brownell SE. 2014. Course-based undergraduate research experiences can make scientific research more inclusive. CBE Life Sci Educ 13:602–606. doi:10.1187/cbe.14-06-0099.
- Brownell SE, Hekmat-Scafe DS, Singla V, Chandler Seawell P, Conklin Imam JF, Eddy SL, Stearns T, Cyert MS. 2015. A high-enrollment course-based undergraduate research experience improves student conceptions of scientific thinking and ability to interpret data. CBE Life Sci Educ 14:ar21. doi:10.1187/cbe.14-05-0092.
- Cooper KM, Knope ML, Munstermann MJ, Brownell SE. 2020. Students who analyze their own data in a course-based undergraduate research experience (CURE) show gains in scientific identity and emotional ownership of research. J Microbiol Biol Educ 21:10.1128/jmbe.v21i3.2157. doi:10.1128/jmbe.v21i3.2157.
- Heller ST, Duncan AP, Moy CL, Kirk SR. 2020. The value of failure: A student-driven course-based research experience in an undergraduate organic chemistry lab inspired by an unexpected result. J Chem Educ 97:3609–3616. doi:10.1021/acs.jchemed.0c00829.
- Lopatto D, Rosenwald AG, DiAngelo JR, Hark AT, Skerritt M, Wawersik M, Allen AK, Alvarez C, Anderson S, Arrigo C, Arsham A, Barnard D, Bazinet C, Bedard JEJ, Bose I, Braverman JM, Burg MG, Burgess RC, Croonquist P, Du C, Dubowsky S, Eisler H, Escobar MA, Foulk M, Furbee E, Giarla T, Glaser RL, Goodman AL, Gosser Y, Haberman A, Hauser C, Hays S, Howell CE, Jemc J, Johnson ML, Jones CJ, Kadlec L, Kagey JD, Keller KL, Kennell J, Key SCS, Kleinschmit AJ, Kleinschmit M, Kokan NP, Kopp OR, Laakso MM, Leatherman J, Long LJ, Manier M, Martinez-Cruzado JC, Matos LF, McClellan AJ, McNeil G, Merkhofer E, Mingo V, Mistry H, Mitchell E, Mortimer NT, Mukhopadhyay D, Myka JL, Nagengast A, Overvoorde P, Paetkau D, Paliulis L, Parrish S, Preuss ML, Price JV, Pullen NA, Reinke C, Revie D, Robic S, Roecklein-Canfield JA, Rubin MR, Sadikot T, Sanford JS, Santisteban M, Saville K, Schroeder S, Shaffer CD, Sharif KA, Sklensky DE, Small C, Smith M, Smith S, Spokony R, Sreenivasan A, Stamm J, Sterne-Marr R, Teeter KC, Thackeray J, Thompson JS, Peters ST, Van Stry M, Velazquez-Ulloa N, Wolfe C, Youngblom J, Yowler B, Zhou L, Brennan J, Buhler J, Leung W, Reed LK, Elgin SCR. 2020. Facilitating growth through frustration: Using genomics research in a course-based undergraduate research experience. J Microbiol Biol Educ 21:10.1128/jmbe.v21i1.2005. doi:10.1128/jmbe.v21i1.2005.
- Chalkowski K, Lepczyk CA, Zohdy S. 2018. Parasite ecology of invasive species: Conceptual framework and new hypotheses. Trends Parasitol 34:655–663. doi:10.1016/j.pt.2018.05.008.
- Doody JS, Green B, Rhind D, Castellano CM, Sims R, Robinson T. 2009. Population-level declines in Australian predators caused by an invasive species. Anim Conserv 12:46–53. doi:10.1111/j.1469-1795.2008.00219.x.
- Potts SG, Biesmeijer JC, Kremen C, Neumann P, Schweiger O, Kunin WE. 2010. Global pollinator declines: Trends, impacts and drivers. Trends Ecol Evol 25:345–353. doi:10.1016/j.tree.2010.01.007.
- Bellard C, Cassey P, Blackburn TM. 2016. Alien species as a driver of recent extinctions. Biol Lett 12:20150623. doi:10.1098/rsbl.2015.0623.
- Powell KI, Chase JM, Knight TM. 2011. A synthesis of plant invasion effects on biodiversity across spatial scales. Am J Bot 98:539–548. doi:10.3732/ajb.1000402.
- Hansen GJ, Vander Zanden MJ, Blum MJ, Clayton MK, Hain EF, Hauxwell J, Izzo M, Kornis MS, McIntyre PB, Mikulyuk A, Nilsson E, Olden JD, Papes M, Sharma S. 2013. Commonly rare and rarely common: Comparing population abundance of invasive and native aquatic species. PLoS One 8:e77415. doi:10.1371/journal.pone.0077415.
- Semenchenko V, Lipinskaya T, Vilizzi L. 2018. Risk screening of non-native macroinvertebrates in the major rivers and associated basins of Belarus using the Aquatic Species Invasiveness Screening Kit. Manag Biol Invasions 9:127–136. doi:10.3391/mbi.2018.9.2.06.
- Moorhouse TP, Macdonald DW. 2015. Are invasives worse in freshwater than terrestrial ecosystems? WIREs Water 2:1–8. doi:10.1002/wat2.1059.
- Griffiths RW, Schloesser DW, Leach JH, Kovalak WP. 1991. Distribution and dispersal of the zebra mussel (Dreissena polymorpha) in the Great Lakes Region. Can J Fish Aquat Sci 48:1381–1388. doi:10.1139/f91-165.
- McMahon RF. 1996. The physiological ecology of the zebra mussel, Dreissena polymorpha, in North America and Europe. Am Zool 36:339–363. doi:10.1093/icb/36.3.339.
- Claudi R, Mackie GL. 1994. Practical manual for zebra mussel monitoring and control. Lewis Publishers, Boca Raton, FL.
- Bastviken DTE, Caraco NF, Cole JJ. 1998. Experimental measurements of zebra mussel (Dreissena polymorpha) impacts on phytoplankton community composition. Freshw Biol 39:375–386. doi:10.1046/j.1365-2427.1998.00283.x.
- Reynolds C, Miranda NAF, Cumming GS. 2015. The role of waterbirds in the dispersal of aquatic alien and invasive species. Divers Distrib 21:744–754. doi:10.1111/ddi.12334.
- Sepulveda AJ, Marczak LB. 2012. Active dispersal of an aquatic invader determined by resource and flow conditions. Biol Invasions 14:1201–1209. doi:10.1007/s10530-011-0149-x.
- Gaston KJ. 2009. Geographic range limits: Achieving synthesis. Proc R Soc B Biol Sci 276:1395–1406. doi:10.1098/rspb.2008.1480.
- Holt RD. 2009. Bringing the Hutchinsonian niche into the 21st century: Ecological and evolutionary perspectives. Proc Natl Acad Sci 106:19659–19665. doi:10.1073/pnas.0905137106.
- Bronnenhuber JE, Dufour BA, Higgs DM, Heath DD. 2011. Dispersal strategies, secondary range expansion and invasion genetics of the nonindigenous round goby, Neogobius melanostomus, in Great Lakes tributaries. Mol Ecol 20:1845–1859. doi:10.1111/j.1365-294X.2011.05030.x.
- Mills EL, Leach JH, Carlton JT, Secor CL. 1993. Exotic species in the Great Lakes: A history of biotic crises and anthropogenic introductions. J Gt Lakes Res 19:1–54. doi:10.1016?S0380-1330(93)71197-1.
- Nakano D, Strayer DL. 2014. Biofouling animals in fresh water: Biology, impacts, and ecosystem engineering. Front Ecol Environ 12:167–175. doi:10.1890/130071.
- Poulin R, Cribb TH. 2002. Trematode life cycles: Short is sweet? Trends Parasitol 18:176–183. doi:10.1016/S1471-4922(02)02262-6.
- Sauer JS, Cole RA, Nissen JM. 2007. Finding the exotic faucet snail (Bithynia tentaculata): Investigation of waterbird die-offs on the Upper Mississippi River National Wildlife and Fish Refuge. U.S. Geological Survey Open-File Report 2007-1065.
- Bachtel RZ, Winter S, Sandland GJ, Koop JAH, Sauer J, Cole RA. 2020. Waterbird mortality monitoring on Navigation pools 7 and 8 of the Upper Mississippi River, 2003–2013. US Fish and Wildlife Service, ServCat 116573.
- Sandland GJ, Houk S, Walker B, Haro RJ, Gillis R. 2013. Differential patterns of infection and life-history expression in native and invasive hosts exposed to a trematode parasite. Hydrobiologia 701:89–98. doi:10.1007/s10750-012-1259-x.
- Sandland GJ, Gillis R, Haro RJ, Peirce JP. 2014. Infection patterns in invasive and native snail hosts exposed to a parasite associated with waterfowl mortality in the upper Mississippi river, USA. J Wildl Dis 50:125–129. doi:10.7589/2013-07-156.
- Bachtel RZ, Rittenhouse M, Sandland GJ, Koop JAH. 2019. Infection patterns of trematodes across size classes of an invasive snail species using field and laboratory studies. Parasitology 146:438–444. doi:10.1017/S0031182018001646.
- Sandland GJ, Peirce JP. 2021. Patterns of Sphaeridiotrema pseudoglobulus infection in sympatric and allopatric hosts (Bithynia tentaculata) originating from widely separated sites across the USA. Parasitol Res 120:187–195. doi:10.1007/s00436-020-06949-0.
- Roy CL, St-Louis V, House J. 2016. Seasonal distribution of the invasive snail, Bithynia tentaculata, within infested waterbodies in Minnesota, USA, including waterfowl migration. Biol Invasions 18:2923–2941. doi:10.1007/s10530-016-1183-5.
- Schock NT, Reisinger AJ, Reisinger LS, Cooper MJ, Cibrowski JJH, Gehring TM, Moerke AH, Ruetz CR, Uzarski DG. 2019. Relationships between the distribution and abundance of the invasive faucet snail (Bithynia tentaculata) and environmental factors in Laurentian Great Lakes coastal wetlands. Biol Invasions 21:2613–2628. doi:10.1007/s10530-019-02000-1.
- Ghosh P. 2021. Generating biological hypotheses in a “Course-based Undergraduate Research Experience” (CURE) course. CourseSource 8. doi:10.24918/cs.2021.3.
- Hoskins SG, Stevens LM, Nehm RH. 2007. Selective use of the primary literature transforms the classroom into a virtual laboratory. Genetics 176:1381–1389. doi:10.1534/genetics.107.071183.
- LaDue ND, McNeal PM, Ryker K, St. John K, van der Hoeven Kraft KJ. 2022. Using an engagement lens to model active learning in the geosciences. J Geosci Educ 70:144–160. doi:10.1080/10899995.2021.1913715.
- Malotky MKH, Mayes KM, Price KM, Smith G, Mann SN, Guinyard MW, Veale S, Ksor V, Siu L, Mlo H, Young AJ, Nsonwu MB, Morrison SD, Sudha S, Bernot KM. 2020. Fostering inclusion through an interinstitutional, community-engaged, course-based undergraduate research experience. J Microbiol Biol Educ 21:10.1128/jmbe.v21i1.1939. doi:10.1128/jmbe.v21i1.1939.
- White KN, Vincent-Layton K, Villarreal B. 2021. Equitable and inclusive practices designed to reduce equity gaps in undergraduate chemistry courses. J Chem Educ 98:330–339. doi:10.1021/acs.jchemed.0c01094.
- Jokinen EH. 1992. The freshwater snails (Mollusca: Gatropoda) of New York State. University of the State of New York, Albany, NY.
Article Files
Login to access supporting documents
Grindle-Bergan-Roller-Johnson-Lunaburg-Koop-Snail Races An Inquiry-Based Approach to Learn Invasive Species Ecology.pdf(PDF | 890 KB)
S1. Snail Races - Instructor Packet.docx(DOCX | 35 KB)
S2. Snail Races - Student Packet.docx(DOCX | 33 KB)
S3. Snail Races - Sample Data Sheet for Analysis.xlsx(XLSX | 18 KB)
S4. Snail Races - Instructor Prelab Presentation.pptx(PPTX | 15 MB)
S5. Snail Races - Student Pre-survey.docx(DOCX | 21 KB)
S6. Snail Races - Student Post-survey.docx(DOCX | 23 KB)
- License terms
Comments
Comments
There are no comments on this resource.